Power System Master Plan Update
Power Cell
Power Division
Ministry of Power, Energy & Mineral Resources
Asian Development Bank
TA No. 4379–BAN: Power Sector Development Program II Component B
Collaborating with:
Bangladesh Power Development Board Power Grid Company of Bangladesh Limited
In Association with: Bangladesh Engineering & Technological Services
June 2006
Contents
Section Page
Acronyms and Abbreviations................................................................................................ ix
Section 1 Executive Summary.......................................................................................... 1-1
1.1 Introduction............................................................................................................. 1-1
1.2 Overview of Power System Planning..................................................................... 1-1
1.3 Summary of Results................................................................................................ 1-3
1.3.1 Existing Power System (Section 2)................................................................. 1-3
1.3.2 Load Forecast (Section 3)............................................................................... 1-8
1.3.3 Fuel Supply (Section 4)................................................................................ 1-10
1.3.4 Generation and Transmission Expansion Options (Section 5)...................... 1-11
1.3.5 Generation Expansion Plan (Section 6)......................................................... 1-16
1.3.6 Transmission Expansion Plan (Section 7)..................................................... 1-25
1.3.7 Economic and Financial Analysis (Section 8)............................................... 1-25
1.4 Recommendations................................................................................................. 1-28
1.4.1 Eliminate Load Shedding.............................................................................. 1-28
1.4.2 Near-Term Strategy....................................................................................... 1-28
1.4.3 Assure Natural Gas Availability................................................................... 1-29
1.4.4 Reduce Uncertainty....................................................................................... 1-29
Section 2 Existing Power System..................................................................................... 2-1
2.1 Overview Of Existing Power System..................................................................... 2-1
2.2 Existing and Committed Generation Projects.......................................................... 2-3
2.3 Existing Transmission System and Committed projects........................................ 2-13
2.3.1 Existing Transmission System...................................................................... 2-13
2.3.2 Committed Transmission Projects................................................................. 2-13
Section 3 Load Forecast.................................................................................................... 3-1
3.1 Introduction............................................................................................................. 3-1
3.2 Load and GDP Analysis......................................................................................... 3-1
3.2.1 Historical Sales and Demand Data.................................................................. 3-1
3.2.2 Historical Net Peak Load................................................................................ 3-3
3.2.3 Load Shedding Data and Analysis.................................................................. 3-3
3.2.4 Distribution of Load by Region...................................................................... 3-4
3.2.5 Historical GDP Data....................................................................................... 3-5
3.2.6 GDP Growth Forecast.................................................................................... 3-6
3.2.7 Demand Side Management, Load Management, and Energy Efficiency......... 3-7
3.3 Forecasting Methodology..................................................................................... 3-10
3.3.1 Regression Analysis..................................................................................... 3-10
3.3.2 Energy Forecasting....................................................................................... 3-11
3.3.3 Peak Demand Forecasting............................................................................. 3-11
3.4 Forecasting Results............................................................................................... 3-12
3.4.1 Electricity Availability By 2020.................................................................... 3-20
3.5 Comparison of Forecasts...................................................................................... 3-20
3.6 Substation Load Forecast...................................................................................... 3-22
3.6.1 Reactive Demand.......................................................................................... 3-22
3.7 Company Load Forecast....................................................................................... 3-23
Section 4 Fuel Supply....................................................................................................... 4-1
Appendices A and B are contained in a separate volume
Table Page
Table 1-1 Generation Capacity by Region............................................................................... 1-6
Table 1-2 Existing Transmission Lines................................................................................... 1-7
Table 1-3 Existing 230/132 KV Transformers........................................................................ 1-7
Table 1-4 Net Energy Generation and Net Peak Load Forecasts............................................. 1-9
Table 1-5 Fuel Price Forecasts.............................................................................................. 1-11
Table 1-6 Generation Expansion Options............................................................................. 1-12
Table 1-7 Sites and Capacity Additions................................................................................ 1-15
Table 1-8 Base Case Unit Additions and System Reliability Indices.................................... 1-19
Table 1-9 Base Case System Costs....................................................................................... 1-20
Table 2-1 Generation Capacity by Region............................................................................... 2-3
Table 2-2 Primary Energy Resources for Existing and Committed Capacity........................... 2-4
Table 2-3 Existing and Committed Generation........................................................................ 2-7
Table 2-4 Rehabilitation Projects........................................................................................... 2-11
Table 2-5 Status of Committed Generation Projects.............................................................. 2-12
Table 2-6 Existing Transmission Lines................................................................................. 2-14
Table 2-7 Existing 230/132 KV Transformers...................................................................... 2-14
Table 2-8 Committed Transmission Projects......................................................................... 2-14
Table 3-1 Historical Sales and Loss Data by Utility................................................................ 3-2
Table 3-2 Net Peak Load Values............................................................................................. 3-3
Table 3-3 Peak Load Analysis................................................................................................ 3-4
Table 3-4 Load Shedding Analysis......................................................................................... 3-4
Table 3-5 Historical Electricity Requirements by Region and Distribution Factors................. 3-5
Table 3-6 Historical GDP and Growth Rates, Constant 1995-6 Taka..................................... 3-6
Table 3-7 GDP Projections and Growth Rates, Constant 1995-6 Taka.................................. 3-7
Table 3-8 Net Energy Generation and Net Peak Load Forecasts........................................... 3-13
Table 3-9 Yearly and Average Growth Rates for Energy and Peak Load............................. 3-13
Table 3-10 Base Case Forecast Net Energy Generation and Net Peak Load by Region........ 3-16
Table 3-11 High Case Forecast Net Energy Generation and Net Peak Load by Region........ 3-17
Table 3-12 Low Case Forecast Net Energy Generation and Net Peak Load by Region........ 3-18
Table 3-13 Forecast Distribution and Trans Losses and Base Case Sales by Region........... 3-19
Table 3-14 Comparison of Forecasts.................................................................................... 3-21
Table 3-15 Demand Forecast by Company........................................................................... 3-24
Table 4-1 Natural Gas Reserves of Bangladesh...................................................................... 4-2
Table 4-2 Natural Gas Production (FY2003).......................................................................... 4-3
Table 4-3 Gas Consumption in FY2003................................................................................ 4-4
Table 4-4 Production Augmentation Projects (FY2005-2010)............................................... 4-4
Table 4-5 Projected Maximum Gas Demand (FY2005-2010)................................................ 4-5
Table 4-6 Gas and Coal Requirement During 2005-2025 (Sufficient Gas Scenario).............. 4-5
Table 4-7 Estimated Coal Reserves......................................................................................... 4-7
Table 4-8 Phulbari Mine Development Timetable................................................................... 4-8
Table 4-9 Historical Fuel Prices.............................................................................................. 4-8
Table 4-10 Fuel Price Forecast.............................................................................................. 4-14
Table 5-1 Generation Expansion Options............................................................................... 5-4
Table 5-2 Summary of Sites and Capacity Additions for Base Case.................................... 5-14
Table 6-1 Cost of Production – Diesel Backup Generation..................................................... 6-5
Table 6-2 Scenarios Evaluated.............................................................................................. 6-10
Table 6-3 Screening Analysis Options.................................................................................. 6-14
Table 6-4 Base Case Unit Additions and System Reliability Indices.................................... 6-24
Table 6-5 Base Case System Costs....................................................................................... 6-25
Table 6-6 Conversion Factors in Calculations of Fuel Usage............................................... 6-27
Table 6-7 Base Case Fuel Use.............................................................................................. 6-28
Table 6-8 Sites and Capacity Addition.................................................................................. 6-29
Table 6-9 High Demand Scenario Unit Additions and System Reliability Indices................ 6-31
Table 6-10 Low Demand Scenario Unit Additions and System Reliability Indices.............. 6-32
Table 6-11 Limited Gas Scenario Unit Additions and System Reliability Indices................. 6-34
Table 6-12 Limited Gas Scenario System Costs................................................................... 6-35
Table 6-13 Limited Gas Scenario Fuel Requirements........................................................... 6-36
Table 6-14 Limited Gas – Early Coal Scenario Unit Additions and System Reliability Indices
...................................................................................................................................... 6-37
Table 6-15 Limited Gas – Early Coal Scenario System Costs.............................................. 6-38
Table 6-16 Limited Gas – Early Coal Scenario Fuel Requirements...................................... 6-39
Table 6-17 Fuel Security Scenario Unit Additions and System Reliability Indices.............. 6-40
Table 6-18 Fuel Security Scenario System Costs................................................................. 6-41
Table 6-19 Fuel Security Scenario Fuel Requirements......................................................... 6-42
Table 6-20 Unit Additions in Coal Scenarios and Base Case............................................... 6-43
Table 6-21 Total Costs by Cost Category in Coal Scenarios and Base Case........................ 6-44
Table 6-22 Study Period Natural Gas and Coal Use in Coal Scenarios and Base Case........ 6-44
Table 6-23 High Disc Rate Scenario Unit Additions and System Reliability Indices........... 6-46
Table 6-24 Low Disc Rate Scenario Unit Additions and System Reliability Indices........... 6-47
Table 6-25 Discount Rate Scenarios Cost Comparison........................................................ 6-47
Table 6-26 Cost of ENS Scenarios Cost Comparison........................................................... 6-49
Table 6-27 No LOLP Criterion Scenarios Cost Comparison................................................ 6-51
Table 6-28 New 500 MW Unit - Cost Savings..................................................................... 6-53
Table 6-29 New 500 MW Unit - Value Summary................................................................ 6-53
Table 6-30 New 1,000 MW Interconnection - Cost Savings................................................ 6-55
Table 6-31 New 1,000 MW Interconnection - Value Summary............................................ 6-56
Table 7-1 Summary of Transmission Planning Criteria.......................................................... 7-4
Table 7-2 Summary Generation Dispatch for All Cases....................................................... 7-10
Table 7-3 Reactive Compensation by Region for 2025......................................................... 7-15
Table 7-4 Generation Load and Losses by Region – Plan A................................................. 7-15
Table 7-5 Short Circuit Currents for Plan A at 2025............................................................. 7-16
Table 7-6 Typical Time Sequence for Dynamic Simulations................................................. 7-17
Table 7-7 Three-Phase Faults................................................................................................ 7-18
Table 7-8 Summary of Transmission Lines for 2025 Transmission Plan A......................... 7-19
Table 7-9 Summary of Transformers for 2025 Transmission Plan A................................... 7-20
Table 7-10 Summary of 2025 Transmission Plan B............................................................. 7-22
Table 7-11 Capacitors 100 MVARS and Larger for Horizon Year 2025............................. 7-22
Table 7-12 Summary Cost Comparison for Plan A and B.................................................... 7-23
Table 7-13 Cost of Components for 2021-2025................................................................... 7-24
Table 7-14 Reactive Compensation by Region for 2010....................................................... 7-30
Table 7-15 Generation Load and Losses by Region 2010..................................................... 7-30
Table 7-16 List of Transmission Lines for 2010................................................................... 7-31
Table 7-17 List of Transformers for 2010............................................................................. 7-31
Table 7-18 Capacitors for 2010 Larger Than 25 MVAR...................................................... 7-32
Table 7-19 Cost of Components for 2005-2010................................................................... 7-33
Table 7-20 Reactive Compensation by Region for 2015....................................................... 7-35
Table 7-21 Generation Load and Losses by Region 2015..................................................... 7-36
Table 7-22 Transmission Lines for Period 2010 to 2015...................................................... 7-36
Table 7-23 Transformers for Period 2010 to 2015................................................................ 7-37
Table 7-24 Capacitors for Period 2010 to 2015.................................................................... 7-37
Table 7-25 Cost of Components for 2015............................................................................. 7-38
Table 7-26 Reactive Compensation by Region for 2020....................................................... 7-41
Table 7-27 Generation Load and Losses by Region 2020..................................................... 7-41
Table 7-28 Transmission Lines for Period 2015 to 2020...................................................... 7-42
Table 7-29 Transformers for Period 2015 to 2020................................................................ 7-43
Table 7-30 List of Capacitors Larger Than 100 MVAR for Year 2020................................ 7-43
Table 7-31 Cost of Components for 2020............................................................................. 7-43
Table 8-1 Unit Class Capacity Factors.................................................................................... 8-5
Table 8-2 Resource Plan Additions for Load Growth Scenarios............................................ 8-7
Table 8-3 Least Cost Options at Different Gas Prices............................................................. 8-9
Table 8-4 Summary of COENS Scenarios............................................................................ 8-10
Table 8-5 Fuel Price Scenarios.............................................................................................. 8-15
Table 8-6 Overall Summary of Cash Flows.......................................................................... 8-15
Table 8-7 Generation Investments......................................................................................... 8-16
Table 8-8 Transmission Investments..................................................................................... 8-17
Table 8-9 Investment Requirements July 2005 through June 2010....................................... 8-18
Figure Page
Figure 1-1 Map of Existing System........................................................................................ 1-5
Figure 1-2 Base, High, and Low Demand Forecasts.............................................................. 1-9
Figure 1-3 All Technologies and Least Cost Curve.............................................................. 1-18
Figure 1-4 Base Case Fuel Use............................................................................................. 1-21
Figure 1-5 Comparison of Costs and Natural Gas Use for Demand Growth Cases............. 1-22
Figure 1-6 Comparison of Total Costs, Base Case vs. Limited Gas Case............................. 1-22
Figure 1-7 Fuel Use in Limited Gas Case vs. Base Case Natural Gas Use........................... 1-23
Figure 2-1 Map of Existing System........................................................................................ 2-2
Figure 3-1 Historical GDP and Net Energy Generation Correlation Graph.......................... 3-11
Figure 3-2 Load Factor Analysis.......................................................................................... 3-12
Figure 3-3 Base Case Energy and Peak Load Forecasts....................................................... 3-14
Figure 3-4 High and Low Case Energy and Peak Load Forecasts........................................ 3-14
Figure 3-5 Comparison of Forecasts..................................................................................... 3-21
Figure 6-1 Cost Representation for Screening Analysis Method.......................................... 6-12
Figure 6-2 Base Load Technologies...................................................................................... 6-15
Figure 6-3 Peaking Technologies.......................................................................................... 6-16
Figure 6-4 Peaking Technologies vs. COENS...................................................................... 6-17
Figure 6-5 All Technologies and Least Cost Curve.............................................................. 6-18
Figure 6-6 Higher Gas Price................................................................................................. 6-19
Figure 6-7 Lower Gas Price.................................................................................................. 6-19
Figure 6-8 Breakeven Coal Price.......................................................................................... 6-20
Figure 6-9 Base Case Fuel Use............................................................................................. 6-27
Figure 6-10 Demand Scenarios - Cost and Fuel Use Comparison........................................ 6-33
Figure 6-11 Total Costs in Coal Scenarios and Base Case.................................................... 6-43
Figure 6-12 Natural Gas and Coal Use in Coal Scenarios and Base Case............................. 6-45
Figure 6-13 Discount Rate Scenarios - Total Installed Capacity........................................... 6-45
Figure 6-14 Cost of ENS Scenarios - Total Installed Capacity.............................................. 6-48
Figure 6-15 Cost of ENS Scenarios - LOLP........................................................................ 6-49
Figure 6-16 No LOLP Scenarios - Total Installed Capacity and Reserve Margins............... 6-50
Figure 6-17 No LOLP Scenarios – Reliability Statistics....................................................... 6-51
Figure 7-1 Horizon Year Development Process..................................................................... 7-7
Figure 7-2 One-Line Diagram of Plan A for Year 2025....................................................... 7-12
Figure 7-3 Stability Plot of NCF-01..................................................................................... 7-19
Figure 7-4 Stability Plot of NCF-04..................................................................................... 7-19
Figure 7-5 One-Line Diagram of Plan A for Year 2010....................................................... 7-27
Figure 7-6 One-Line Diagram of Plan A for Year 2015....................................................... 7-34
Figure 7-7 One-Line Diagram of Plan A for Year 2020....................................................... 7-39
Figure 8-1 Least Cost Curve – Base Case............................................................................... 8-2
Figure 8-2 Comparison of Peak Load Technologies.............................................................. 8-3
Figure 8-3 Energy Not Served and Equivalent Capacity Factor.............................................. 8-5
Figure 8-4 Least Cost Curve – High Gas Prices..................................................................... 8-8
Figure 8-5 Least Cost Curve – Low Gas Prices...................................................................... 8-9
Figure 8-6 LOLP Expectation for Cost of ENS Cases.......................................................... 8-11
Acronyms and Abbreviations
AC | Alternating Current |
ADB | Asian Development Bank |
AECB | Asia Energy Corporation Bangladesh |
BAPEX | Bangladesh Petroleum Exploration and Production Company Limited |
BDT | Bangladesh Taka |
BETS | Bangladesh Engineering & Technological Services |
BOGMC | Bangladesh Oil, Gas, and Mineral Corporation (Petrobangla) |
BPDB | Bangladesh Power Development Board |
BSCF | Billion Standard Cubic Feet |
CC | Combined cycle |
COENS | Cost of energy not served |
DC | Direct Current |
DESA | Dhaka Electric Supply Authority |
DESCO | Dhaka Electric Supply Company Limited |
EIA | Energy Information Agency |
ENS | Energy Not Served |
FY | Fiscal Year |
GDP | Gross Domestic Product |
GJ | Gigajoule |
GOB | Government of Bangladesh |
GSB | Geological Survey of Bangladesh |
GWH | Gigawatt Hour |
IAEA | International Atomic Energy Agency |
IDC | Interest During Construction |
IOC | International Oil Company |
IPP | Independent Power Producer |
KA | Kiloampere |
KCAL | Kilocalorie |
KJ | Kilojoule |
KM | Kilometer |
KVA | Kilovolt Ampere |
KVAR | Kilovolt Ampere Reactive |
KW | Kilowatt |
KWH | Kilowatt Hour |
LOLP | Loss of Load Probability |
MJ | Megajoule |
MMSCFD | Million Standard Cubic Feet per Day |
MVA | Megavolt Ampere |
MVAR | Megavolt Ampere Reactive |
MW | Megawatt |
MWH | Megawatt Hour |
NPV | Net Present Value |
OPEC | Organization of Petroleum Exporting Countries |
ORNL | Oak Ridge National Laboratory |
O&M | Operation and Maintenance |
PBS | Palli Budyet Samity (a form of rural electric cooperative) |
PCP | Project Concept Paper |
PGCB | Power Gird Company of Bangladesh Limited |
PMU | Project Management Unit |
PP | Project Pro-forma |
PSC | Production Sharing Contract |
PSMP | Power Sector Master Plan |
REB | Rural Electrification Board |
SCF | Standard Cubic Foot |
SCGT | Simple Cycle Gas Turbine |
TA | Technical Assistance |
TSCF | Trillion Standard Cubic Feet |
TMT | Thousand Metric Tonnes |
TOR | Terms of Reference |
TVA | Tennessee Valley Authority |
UK | United Kingdom |
US | United States |
WASP | Wien Automatic System Planning package |
WZPDCL | West Zone Power Distribution Company Limited |
Section 1 Executive Summary
1.1 INTRODUCTION
Nexant, Inc. has been retained by the Asian Development Bank (ADB), under Contract No. COCS/05-158, to provide consulting services for the Power Sector Development Program II study. The objective of this Technical Assistance (TA) assignment is to assist the Government of Bangladesh in preparing the Power Sector Development Program II and to support the policy reform and further restructuring of the power sector. A key part (Component B) of the TA is to develop a Power System Master Plan (PSMP) Update to the year 2025. This effort completely revises the 1995 Power System Master Plan, the most recent previous update.
Nexant assisted Bangladesh Power Development Board, Power Gird Company of Bangladesh Limited, and Power Cell in developing this PSMP Update.
Section 1.2 elaborates on the objectives of the PSMP update and provides an overview of power system planning in general. Section 1.3 summarizes the results of the PSMP Update. Section 1.4 offers our recommendations. The rest of this report is organized as shown below, generally following the content and sequence outlined in Section 1.2.
- Section 2 provides an overview of the existing generation and transmission systems.
- Section 3 presents our load forecast.
- Section 4 addresses energy resources, especially fuel supply. .
- Section 5 discusses generation and transmission expansion options and potential generating plant sites.
- Section 6 provides the generation expansion plan.
- Section 7 presents the transmission expansion plan.
- Section 8 discusses economic analysis and financial projections for the proposed development plan.
1.2 OVERVIEW OF POWER SYSTEM PLANNING
Power system planning in Bangladesh must be consistent with the Government of Bangladesh’s (GOB’s) objectives for the power sector. The GOB’s 1994 paper “Power Sector Reforms in Bangladesh” outlined a reform process focusing on institutional issues. The 2000 Vision and Policy Statement for the Power Sector Reforms elaborated on institutional issues for the power sector. It also gave specific direction on issues related to the physical planning of the power system, of which the most relevant for power system planning are:
- Make electricity available for all by 2020
− The load forecast and generation and transmission expansion plans need to account for this.
- Ensure reliable and quality supply of electricity
− An appropriate level of reliability is a specific target of the planning process.
- Increase the sector's efficiency
− New power plants are the most efficient, cost effective of their type. The planning process adds enough generation and transmission to provide cost- effective reliability.
- Develop demand management and energy efficiency measures
− The load forecast needs to account for this.
- Develop alternative/renewable energy sources
− The new generation options need to address this.
- Base new generation on a least cost expansion plan
− This is the basis for the generation expansion planning procedures.
- Expand transmission in balance with the generation capacity
− This is the basis for the transmission expansion planning procedures.
In summary, the planning process achieves these objectives if it is done comprehensively and based on least cost principles. One fundamental trade-off is between cost and reliability. The planning process undertaken in this study quantifies the value of reliability. It balances the benefit of increased reliability against the cost of achieving it, and adds new generation until that balance is achieved.
The planning process proceeds roughly in the sequence shown below.
− Planning criteria, description of the existing system, fuel supply and costs, economic data, existing plant cost, performance, rehabilitation, and retirements, expansion options and sites
− Analysis of historical data, approach, energy forecast, capacity forecast, regional demand, substation loads
- Step 3. Fuel supply forecast
− Availability and costs of different fuels
- Step 4. Generation expansion plan
− Prepare inputs; conduct screening analysis; analyze thousands of alternative plans to get least-cost option that meets criteria; summarize key outputs such as type, fuel, timing, and size of new generating units, the output and fuel use of all generating units, the costs of all cost categories, reliability statistics, etc.
− Identify sites for the generating plants and place specific new plants on those sites in specific years for transmission planning purposes.
- Step 6. Transmission expansion plan
− Prepare inputs; establish pattern of generation for each study year; conduct load flows, stability, short circuit, losses, and cost analyses; identify transmission plan that best meets planning and cost objectives; summarize the type, timing, size, of new transmission facilities
The completion of the generation and transmission expansion plans typically represents the end of the power system planning process. The Terms of Reference for Component B include separate tasks for an environmental assessment and a financial and economic analysis. These might be considered to be the beginning of the implementation process that leads to project design, construction, and operation. Section 8 of this report addresses the financial and economic assessment. A separate document, Volume 3 of the Component C report, provides among other things the environmental assessment.
1.3 SUMMARY OF RESULTS
The data and results in this report are generally expressed in terms of fiscal years, which for the Bangladesh power sector organizations end in on 30 June. For example, the fiscal year 2003 runs from 1 July 2002 to 30 June 2003.
1.3.1 Existing Power System (Section 2)
1.3.1.1 Overview of Existing Power System
Demand in Bangladesh is concentrated in the Dhaka region, which had 47% of the national total of 3,952 MW (including estimated load shedding) in 2004. Significant load shedding due primarily to lack of generation occurred in each of the historical years reviewed, reaching 461 MW at time of peak in 2004.
Natural gas from fields in the eastern part of the country fuels the vast majority of the existing power plants.
Placing power plants close to Dhaka minimized transmission costs and losses, and helped maintain voltages. Dhaka is also relatively accessible from the eastern gas fields. Thus in general terms the generation system today consists of multiple plants with 61% of national capacity near Dhaka, with smaller plants near the gas fields or other load centers.
The highest voltage transmission system consists of a 230 KV loop around Dhaka with radial extension to the other regions. The 132 KV system initially extended radially from Dhaka to the other regions, but now includes loops ringing Dhaka and Chittagong, and larger loops in the Southern, Western, and Northern regions.
Figure 1-1 provides a map of the existing generation and transmission systems that also shows the main natural gas fields.
1.3.1.2 Existing and Committed Generation Projects
Table 1-1 summarizes generation by region. As of June 2004 existing net capacity is 4,120 MW. We include “committed” generation of 2,845 MW as well as existing plants in the planning process. These are plants that are not yet operational, but that are far enough along in the process of approving, financing, and building that it is highly likely that they will be built and become operational. In other words, these are plants that are not subject to being displaced by new units that the generation planning process may identify.
Delays in completing the rehabilitation projects or especially the committed projects will reduce the amount of generation available to meet load in the first few years of the planning period. Thus is especially important to complete these projects, because in many cases there is not enough time to replace their generation with new plants. The current status indicates that most of the projects are progressing towards completion on or close to schedule.
However, in some cases funding has not been completed. Many of the projects rely on GOB funding, for which there may be competing needs.
Figure 1-1 Map of Existing System
Table 1-1 Generation Capacity by Region
Region | Dhaka | Central | Southern | Northern | Western | National |
Number of Existing Units June 2004 | 23 | 11 | 4 | 4 | 16 | 58 |
Existing Generation June 2004, Derated Net MW | 2,506 | 345 | 605 | 260 | 404 | 4,120 |
% of National Total MW | 61% | 8% | 15% | 6% | 10% | 100% |
MW/Unit | 109 | 31 | 151 | 65 | 25 | 71 |
Number of Committed Units | 8 | 3 | 2 | 5 | 1 | 19 |
Committed Generation to 2009, Derated Net MW | 1,342 | 257 | 199 | 850 | 197 | 2,845 |
% of National Total MW | 47% | 9% | 7% | 30% | 7% | 100% |
Number of Existing and Committed Units | 31 | 14 | 6 | 9 | 17 | 77 |
Total Existing and Committed, Derated Net MW | 3,848 | 602 | 804 | 1,110 | 601 | 6,965 |
% of National Total MW | 55% | 9% | 12% | 16% | 9% | 100% |
1.3.1.3 Existing Transmission System and Committed Projects
Figure 1-1 shows PGCB’s transmission system. This figure includes all the existing 230 KV and 132 KV transmission lines as solid lines and planned and under construction facilities as dashed lines.
The highest voltage level of PGCB’s transmission system is 230 KV with transmission lines structured as a 230 KV loop around Dhaka with radial extension to the western part of Bangladesh and the Southern Region in the Chittagong area. The 132 KV system extends radially from Dhaka to the Central and Southern regions. Similarly, the Northern and Western regions in the western part of the country are interconnected through 132 KV lines.
Table 1-2 summarizes the existing transmission lines. Table 1-3 summarizes the 230/132 KV transformers.
The paragraphs above summarize the structure of the present transmission system. This system currently experiences problems of low voltages, not only in the Dhaka area, but also in the other regions of the country. PGCB is well aware of these problems and has taken steps to initiate a program of reactive compensation by way of adding shunt capacitors at different locations of the transmission system.
Table 1-2 Existing Transmission Lines
Region | Voltage, Nominal kV | Length, Circuit-Km |
Southern | 230 | 623 |
| 132 | 1326 |
Dhaka | 230 | 673 |
| 132 | 597 |
Central | 132 | 804 |
Western | 230 | 140 |
| 132 | 990 |
Northern | 132 | 1151 |
Total | 230 | 1436 |
| 132 | 4868 |
Table 1-3 Existing 230/132 KV Transformers
Region | Capacity (MVA) |
Southern | 675 |
Dhaka | 2800 |
Northern | 450 |
Total | 3925 |
Currently there are several transmission projects under construction. A second 230 KV line that will interconnect the eastern and western portions of the country is among the most important, contributing to a significant improvement of PGCB’s transmission system reliability. This line will go through the Jamuna Bridge from the Ashuganj substation in the eastern part of the country to the Sirajganj substation in the west. Another 230 KV line is under construction from Barapukuria in the upper part of the Northern region of Bangladesh to Sirajganj near the Jamuna River. Similarly a 230 KV line is under construction from Ishurdi in the northern region to Khulna in the Western region of Bangladesh.
There are several committed projects in the Dhaka area. One of them is a committed 400 kV double circuit line that will go from the area around the existing Meghnaghat 230 kV substation to the existing Aminbazar 230 kV substation and it is expected to become operational by 2010. This line though insulated at 400 kV will initially be operated at 230 kV. It is expected that this line will start operation at 400 kV in the year 2015. Another committed project is a transmission line from the existing Aminbazar 230 kV substation to a new Old Airport 230 kV substation. This line is a double circuit transmission facility that will assist in supporting the load growth in the western part of Dhaka. There is also a committed double circuit transmission facility to go from Ullon 132 kV substation to the Rampura 132 kV substation.
There are several committed projects in other regions of the country as well. In the Western region two double circuit 132 kV lines are expected to be built from the 132 kV Jhenida substation to new 132 kV substations at Magura and Chuadanga. In the Northern region two double circuit 132 kV lines are expected to be built, one from the Naogaon 132 kV substation to a new 132 kV substation at Joypurhat and another from the Thakurgaon 132 kV substation to a new 132 kV substation in Panchaghar.
1.3.2 Load Forecast (Section 3)
Over the last ten years net energy demand growth at an average compound annual rate of 8.1% has accompanied gross domestic product (GDP) growth at an average of 5.1%.
Electricity demand has grown at about the same rate in all regions. Load factor has been steady but slightly increasing. Load shedding has been a problem throughout the period. The Rural Electrification Board (REB), and then DESCO, have picked up substantial parts of what was previously DESA and BPDB loads. Distribution losses have dropped from an average of 30% in 1994 to 21% in 2004. Transmission losses fell from 4.7% to 3.5% during the same period.
The demand forecast is based on the excellent historical correlation of electricity demand with GDP, and three forecasts of GDP growth through 2025. The Base Case uses GDP figures whose compound average annual growth rate is 5.2%. The Low Case GDP figures average annual rate is 4.5%. The High Case is based on a GOB forecast with an annual average rate of 8.0%.
These GDP growth rates produce net energy demand growth rates to 2025 of 7.9% for the Base Case, 6.7% for the Low Case, and 12.0% for the High Case. Figure 1-2 and Table 1-4 present these forecasts in graphical and tabular format. These are grid input values, referred to the high voltage side of power plant main transformers. We have assumed that transmission and distribution losses continue to fall. For transmission, they drop to 3.0% by 2018. Distribution losses drop to 10% by 2019. In our approach the impact of these loss reductions is to increase forecast sales, which then grow slightly faster than net energy generation in the future as they have in the past. In the Base Case sales grow at an annual average 8.5% compared to 7.9% for net energy generation.
We compared this study’s forecast with the forecast developed for the 1995 Power System Master Plan (1995 PSMP), the recent forecast developed as part of the current ADB Gas Development Project, and the recent forecast developed as part of the 2005 Gas Sector Master Plan.. All four forecasts are very close; the ADB Gas study would be even closer if the gross generation figures were reduced to correspond to the net values used in the other three studies. This confirms earlier observations that 1995 PSMP load forecast was very accurate, and also demonstrates that the calculations for natural gas use in the power sector in the ADB Gas study and in the 2005 Gas Sector Master Plan study are based on an electricity generation forecast similar to the Base Case of this study.
Based on the regional distribution of demand reduced to account for transmission losses, we developed demand forecasts for each transmission substation expected to be in place by 2010. Based on our analysis of historical trends, we forecast that demand would grow at the same rate in each region as nationally. We did not constrain individual substations to grow at this rate. We did require that the total substation demand in each region equal the forecast regional demand. Individual substations in a region were assigned higher or lower growth rates based on PGCB’s view of likely differences in growth rates in different areas.
Consistent with a review of 2005 data and also based on discussions with BPDB and PGCB, this study forecasts reactive demand at each transmission substation based on a power factor of 90% for the transmission analysis study years 2010, 2015, 2020,and 2025.
| Base Case | High Case | Low Case | Projected Load Factor | Fiscal Year | Net Generation (GWh) | Net Peak Load (MW) | Net Generation (GWh) | Net Peak Load (MW) | Net Generation (GWh) | Net Peak Load (MW) | 2005 | 21,964 | 4,308 | 22,336 | 4,381 | 21,964 | 4,308 | 58.2% | 2006 | 23,945 | 4,693 | 24,692 | 4,839 | 23,611 | 4,627 | 58.2% | 2007 | 26,106 | 5,112 | 27,297 | 5,345 | 25,382 | 4,970 | 58.3% | 2008 | 28,461 | 5,569 | 30,177 | 5,904 | 27,286 | 5,339 | 58.3% | 2009 | 31,028 | 6,066 | 33,592 | 6,567 | 29,333 | 5,734 | 58.4% | 2010 | 33,828 | 6,608 | 37,652 | 7,355 | 31,533 | 6,160 | 58.4% | 2011 | 36,622 | 7,148 | 42,202 | 8,237 | 33,659 | 6,569 | 58.5% | 2012 | 39,647 | 7,732 | 47,627 | 9,288 | 35,928 | 7,007 | 58.5% | 2013 | 42,922 | 8,364 | 53,749 | 10,473 | 38,351 | 7,473 | 58.6% | 2014 | 46,467 | 9,047 | 60,659 | 11,810 | 40,937 | 7,970 | 58.6% | 2015 | 50,306 | 9,786 | 68,924 | 13,408 | 43,697 | 8,501 | 58.7% | 2016 | 54,079 | 10,512 | 78,316 | 15,223 | 46,643 | 9,066 | 58.7% | 2017 | 58,135 | 11,291 | 88,384 | 17,166 | 49,788 | 9,670 | 58.8% | 2018 | 62,496 | 12,128 | 99,746 | 19,357 | 53,145 | 10,313 | 58.8% | 2019 | 67,183 | 13,027 | 112,568 | 21,827 | 56,728 | 11,000 | 58.9% | 2020 | 72,222 | 13,993 | 126,172 | 24,445 | 60,553 | 11,732 | 58.9% | 2021 | 77,092 | 14,924 | 141,419 | 27,377 | 64,178 | 12,424 | 59.0% | 2022 | 82,290 | 15,917 | 158,510 | 30,661 | 68,020 | 13,157 | 59.0% | 2023 | 87,839 | 16,977 | 176,448 | 34,103 | 72,092 | 13,934 | 59.1% | 2024 | 93,761 | 18,107 | 196,415 | 37,931 | 76,408 | 14,756 | 59.1% | 2025 | 100,083 | 19,312 | 217,137 | 41,899 | 80,982 | 15,626 | 59.2% |
| |
Figure 1-2 Base, High, and Low Demand Forecasts Table 1-4 Net Energy Generation and Net Peak Load Forecasts
1.3.3 Fuel Supply (Section 4)
Presently, natural gas is the only significant source of commercial energy in Bangladesh. About 85% of the power generation capacity in the country is gas based, 10% is imported oil based and 5% hydro. About 90% of electrical energy is generated by natural gas. Barapukuria Coal Mine in the north-west region of Bangladesh will supply coal to BPDB's first coal based 2x125 MW power plant, now under construction, by FY2006. There are also very good prospects of extraction of coal from a nearby coal deposit at Phulbari, and possibly elsewhere in the region.
Bangladesh Oil, Gas and Mineral Corporation (BOGMC, also known as Petrobangla) is the state-owned monopoly supplier of power plant fuels and is responsible for most fuels activity in Bangladesh. Petrobangla is under the administrative control of the Energy and Mineral Resources Division of the Ministry of Power, Energy and Mineral Resources.
Present gas reserves and production are adequate to serve the existing power system. It is highly probable that additional reserves could be developed to serve the needs of the power plants projected in the Master Plan. It is also likely that substantial coal could be developed in the area of current coal development. A planned 100 MW expansion of the existing hydro plant is the only substantial additional hydro feasible in the country. Imported coal or petroleum products are the other main options for fuel supply.
We base our fuel price forecasts on world market prices for petroleum liquids and coal. We base the forecast on an opportunity cost approach for natural gas, pricing it at 75% of the forecast price of heavy fuel oil, on an equivalent GJ basis. We understand that some of Petrobangla’s Production Sharing Contracts use 75% of a fuel oil benchmark as the key part of the calculation of price. The source data are crude oil and coal price forecasts from the US Energy Information Agency’s (EIA’s) Annual Energy Report 2005. EIA characterizes its forecasts as being of world market prices, in other words not specific to just the US. Both coal and especially oil are traded extensively in world markets. Thus prices in any open market situation should relate to their world market prices.
Table 1-5 provides the resulting prices, levelized in constant dollars over 2005 - 2025. We estimate fuel oil prices to be 75% of crude oil prices. This is based on historical data for the ratio of fuel oil price to crude oil price in dollars per GJ.
A market for domestic coal may develop in Bangladesh, given that several fields have been identified and more are possible. However, no realistic market exists today, so we must estimate prices using another approach. The key factor in the domestic prices shown in Table 1-5 is establishing the domestic fuel price at 80% of the price of imported coal, on a $/ton basis. The following concepts led us to this approach.
- Because coal is a world market product, domestic coal will have to compete with imported coal to supply power plants. Unless it is subsidized or protected from competition, it should cost no more than imported coal.
- If coal could be produced for much less than 80% as much as the cost of imported coal, it might well be economic to export it rather than use it domestically. In other words the world market price would influence the price of domestic coal regardless of its cost of production, unless the coal facility were required to subsidize other activities.
- The cost of coal, and to some extent its price, will vary substantially among mines, so no single price can be accurate for all mines. We understand that the Phulbari Coal Project may be able to supply its coal for less than the estimated forecast cost of imported coal, whereas the cost of coal from the Barapukuria Coal Mine may be higher.
Table 1-5 Fuel Price Forecasts
Fuel | Units | 2005 | 2010 | 2015 | 2020 | 2025 | | Level- ized | | Levelized in US cents per million Kcal |
Crude Oil Price (2003 US$) 1 | $/BBL | 33.99 | 25.00 | 26.75 | 28.50 | 30.31 | | 28.23 | |
Crude Oil price (2005 US$) | $/GJ | 6.47 | 4.76 | 5.09 | 5.42 | 5.77 | | 5.37 | | |
Heavy Fuel Oil Price 2 | $/GJ | 4.85 | 3.57 | 3.82 | 4.07 | 4.33 | | 4.03 | | 1686 |
High Sulfur Diesel 3 | $/GJ | 7.44 | 5.47 | 5.85 | 6.24 | 6.63 | | 6.18 | | 2585 |
Natural Gas Price 4 | $/GJ | 3.64 | 2.68 | 2.86 | 3.05 | 3.24 | | 3.02 | | 1265 |
Imported Coal 5 | $/Ton | 41.33 | 39.29 | 37.40 | 37.20 | 36.06 | | 39.56 | | |
Imported coal incl. transport | $/Ton | 56.45 | 54.28 | 52.28 | 52.07 | 50.85 | | 54.72 | | |
Imported Coal | $/GJ | 2.26 | 2.17 | 2.09 | 2.08 | 2.03 | | 2.19 | | 916 |
Domestic coal 6 | $/Ton | 45.16 | 43.43 | 41.82 | 41.66 | 40.68 | | 43.78 | | |
Domestic coal | $/GJ | 1.96 | 1.89 | 1.82 | 1.81 | 1.77 | | 1.90 | | 797 |
Notes
1. EIA 2005 Energy Outlook (price at refineries)
2. Fuel oil price is 0.75 of the forecast crude oil price.
3. LS diesel 1.20, and high sulfur diesel 1.15 for fuel oil price
4. Natural gas price is 0.75 of estimated fuel oil price
5. EIA 2005 Energy Outlook (exported price FAS of US coal)
6. Assumes 80% of Imported coal cost in $/Ton
1.3.4 Generation and Transmission Expansion Options (Section 5)
1.3.4.1 Generation
Fuel availability and cost drive the selection of generation options. Bangladesh has substantial proven reserves of natural gas and some proven reserves of coal. Both are available at reasonable cost. There is reason to expect that more natural gas and coal reserves will be discovered as exploration continues.
Bangladesh’s limited hydro potential will have been completely developed when the 100 MW extension of the existing Karnafuli plant is completed. Thus additional hydro capacity is not a feasible option for additional units.
Although Bangladesh has had some successes in small-scale development of renewable resources, they are peripheral to the issue of generating bulk electricity for the main grid. We have not found any renewable resource generating options likely to be economic for that purpose.
Table 1-6 summarizes the cost and performance characteristics for the selected generation technology options.
Section 1 Executive Summary
Table 1-6 Generation Expansion Options
| | | | | | | | | Net Heat Rates at Grid, KCAL/KWH | | O&M Cost |
Unit Type | Net Unit Capacity (At Grid) | Thermal Parameters | Equipment Cost $/kW | Installation Cost $/kW | Total Project Cost $/kW | Construc-tion Time Per Unit, Months | Plant Life, Years | Minimum Load, % of Capacity | At 100% Power | At 75% Power | At 50% Power | Weeks/year Sched Maint | FOR, % | Fixed $/kW-month | Vari-able $/MWH |
Steam (coal) with FGD | 300 | Note 1 | 1,097 | 269 | 1,366 | 60 | 30 | 50% | 2,173 | 2,217 | 2,287 | 8 | 8% | 0.58 | 1.80 |
Steam (coal) with FGD | 500 | Note 1 | 896 | 215 | 1,111 | 60 | 30 | 50% | 2,154 | 2,198 | 2,268 | 8 | 8% | 0.58 | 1.80 |
CC (natural gas) | 300 | Dual Pressure | 438 | 232 | 670 | 36 | 25 | 50% | 1,720 | 1,856 | 2,023 | 6 | 6% | 0.42 | 2.00 |
CC (natural gas) | 450 | Triple Pressure | 361 | 232 | 593 | 36 | 25 | 50% | 1,686 | 1,819 | 1,984 | 6 | 6% | 0.38 | 1.80 |
CC (natural gas) | 700 | Triple Pressure | 322 | 180 | 502 | 36 | 25 | 50% | 1,564 | 1,688 | 1,840 | 6 | 6% | 0.38 | 1.80 |
SCGT (natural gas) | 100 | | 238 | 163 | 401 | 24 | 20 | 50% | 2,687 | 2,986 | 3,161 | 4 | 4% | 0.42 | 2.50 |
SCGT (natural gas) | 150 | | 227 | 122 | 349 | 24 | 20 | 50% | 2,605 | 2,894 | 3,064 | 4 | 4% | 0.42 | 2.50 |
Steam (natural gas) | 300 | Note 1 | 711 | 263 | 974 | 60 | 30 | 50% | 2,127 | 2,171 | 2,239 | 6 | 6% | 0.58 | 1.60 |
Steam (natural gas) | 500 | Note 1 | 579 | 211 | 789 | 60 | 30 | 50% | 2,109 | 2,152 | 2,220 | 6 | 6% | 0.58 | 1.60 |
Nuclear (light water reactor) | 500 | Note 2 | 1,739 | 978 | 2,717 | 60 | 40 | 50% | 2,598 | 2,651 | 2,735 | 6 | 8% | 1.67 | 0.50 |
Diesel (diesel fuel) | 10 | Medium Speed | | | 450 | 24 | 15 | 50% | 2,900 | 3,050 | 3,200 | 3 | 15% | 0.83 | 3.00 |
| | | | | | | | | | | | | | | |
Note 1: Superheat, Single Reheat, 165 bar/538 deg C/538 deg C | | | | | | | | | |
Note 2: Saturated, Steam Reheat, 73 bar/293 deg C | | | | | | | | | | | |
| | | | | | | | | | | | | | | |
1.3.4.2 Transmission
For transmission options, the focus is on amount of power to transfer, distance to transfer, and the continuing reliability of the interconnected system. In broad strokes, it is possible to assess what transmission options should be considered by conducting network analyses of far future or horizon year conditions. For instance, by identifying major pockets of load and how demand growth will be supplied to each load pocket, it is possible to determine the voltage level for future transmission expansion. Based on the analysis, 750 KV development is far beyond the needed capacity, while 132 KV will require many circuits to support the future growth of Dhaka. Hence, the transmission options are 230 KV and 400 KV for future supply of the Dhaka region.
For the Master Plan study, the expansion options comprise:
- 400 KV AC lines: 4x795 MCM ACSR conductors per phase
- 230 KV AC lines: 2x795 MCM AAAC conductors per phase
- 132 KV AC lines: 636 MCM AAAC conductor per phase
For congested urban areas such as Dhaka, where new right-of-way might be difficult or impossible to obtain, voltage uprating is a potential development option. In this option, the existing 132 KV line is retained, with modifications to allow for operation at 230 KV. In practice, the feasibility of the uprate will depend on specific characteristics of each uprated line. For planning purposes, we assume that the uprate is feasible. If it does turn out to be infeasible, the alternative is to replace the existing 132 KV line with a new 230 KV line on the same right-of-way. There is an additional cost impact to this that would need to be considered at time of implementation.
The options above did not include others that might be considered in a similar Master Plan study for specific reasons.
- High-voltage DC lines. The terminal costs for these lines tend to be relatively high, and thus these lines are economically viable where the application is for distances of 200 KM or more for transfers of 1,000 MW or more.
- Other voltage levels such 500 or 345 KV. The typical approach is to double the voltage level to take advantage of economies of scale. However, at 500 KV, we would have more capacity than needed for the horizon year, and at 345 KV, not enough capacity. The 400 KV level was a reasonable choice for next voltage level to include amongst the options.
For transformers, we aimed to standardize future additions to the following sizes:
- For 230/132 KV transformers: 225/225 MVA comprising of 3 single-phase units
- For 400/230 KV transformers: 375/375 MVA comprising of 3 single-phase units
Where existing transformers of different capacity were at the substations, we considered as an option adding future transformers of same capacity as the existing units. This is to avoid loading imbalance and circulating currents on the transformers when they operate in parallel.
1.3.4.3 Generation Sites
We selected thermal power plant sites based on a number of factors, including:
- Proximity to the load centers and their forecast load demand.
- Transmission to the load centers.
- Availability of adequate space at the site.
- The value of the land for other uses.
- The suitability of the ground and geotechnical conditions for construction of the plant.
- The possibility of flooding or seismic events.
- Potential sources of cooling water and makeup water.
- Fuel deliverability at the site.
- The impact of the facility in a positive or negative manner on the local environment.
- Sources of fill and construction materials.
- Access to the site for transportation of heavy equipments and construction materials.
- Availability of social facilities near site.
We established site rankings based on consideration of the above factors. Using these factors, we identified sites capable of accommodating the capacity of all committed and planned new units, a total of 20,495 MW. The generation planning process described in Section 6 provided the timing, technology, and size of the new units for the Base Case. We placed the units determined by that process at the sites listed in Table 1-7.
Table 1-7 Sites and Capacity Additions
Plant (Com = Committed) | Region | Unit Type | Unit # | Actual Year of Operation | Fuel | Net Capac, MW |
Gorasal Unit 1 Com (Under Maintenance) | Dhaka | ST | 1 | 2005 | Gas | 37 |
Tongi Com | Dhaka | CT | 1 | 2005 | Gas | 104 |
Sidhirganj Com | Dhaka | ST | 1 | 2005 | Gas | 197 |
Mymenshing RPC Com (CC Conv) | Central | ST/CC | 1 | 2006 | Gas | 70 |
Barapukuria Coal Com | Northern | ST | 1 | 2006 | Dom Coal | 115 |
Barapukuria Coal Com | Northern | ST | 2 | 2006 | Dom Coal | 115 |
Baghabari Barge Mtd Com (CC Conv) | Northern | ST/CC | 1 | 2006 | Gas | 40 |
Sidhirganj Com | Dhaka | CT | 1 | 2007 | Gas | 119 |
Sidhirganj Com | Dhaka | CT | 2 | 2007 | Gas | 119 |
Sidhirganj Com | Dhaka | CT | 3 | 2007 | Gas | 119 |
Sylhet Com | Central | CT | 1 | 2007 | Gas | 99 |
Chandpur Com | Southern | CC | 1 | 2007 | Gas | 99 |
Baghabari Barge Mtd Com | Northern | CC | 1 | 2007 | Gas | 130 |
Fenchuganj Com | Central | CC | 1 | 2008 | Gas | 88 |
Meghnaghat Com | Dhaka | CC | 1 | 2008 | Gas | 450 |
Sidhirganj Com | Dhaka | ST | 2 | 2009 | Gas | 197 |
Karnafuli Hydro HY2 Com | Southern | HY | 1 | 2009 | Hydro | 100 |
Khulna ST#2 Com | Western | ST | 2 | 2009 | Gas | 197 |
Siraganj Com | Northern | CC | 1 | 2009 | Gas | 450 |
Haripur | Dhaka | CC | 1 | 2008 | Gas | 150 |
Sikalbaha | Southern | CT | 1 | 2008 | Gas | 150 |
Bogra | Northern | CT | 1 | 2008 | Gas | 150 |
Bhola | Western | CC | 1 | 2008 | Gas | 150 |
Meghnaghat | Dhaka | CC | 2 | 2009 | Gas | 450 |
Khulna | Western | CT | 1 | 2009 | Gas | 100 |
Sikalbaha | Southern | CC | 1 | 2010 | Gas | 450 |
Meghnaghat | Dhaka | CC | 3 | 2011 | Gas | 450 |
Sylhet | Central | CT | 1 | 2011 | Gas | 150 |
Sirajganj | Northern | CC | 2 | 2012 | Gas | 450 |
Bheramara | Western | CC | 1 | 2012 | Gas | 450 |
Haripur | Dhaka | CT | 1 | 2013 | Gas | 150 |
Madanhat/New Sikalbaha | Southern | CC | 1 | 2013 | Gas | 450 |
Amibazar/Dhaka West | Dhaka | CC | 1 | 2014 | Gas | 450 |
Madanhat/New Sikalbaha | Southern | CC | 2 | 2014 | Gas | 450 |
Siddhirganj | Dhaka | CC | 1 | 2015 | Gas | 450 |
Shahjbazar | Central | CT | 1 | 2015 | Gas | 150 |
Khulna | Western | CC | 1 | 2015 | Gas | 450 |
Amibazar/Dhaka West | Dhaka | CC | 2 | 2016 | Gas | 450 |
Rajshanj | Northern | CC | 1 | 2016 | Gas | 450 |
Ashuganj | Dhaka | CT | 1 | 2017 | Gas | 150 |
Amibazar/Dhaka West | Dhaka | CT | 1 | 2017 | Gas | 150 |
Sylhet | Central | CT | 2 | 2017 | Gas | 150 |
Mymensingh New Site | Central | CT | 1 | 2017 | Gas | 150 |
Madanhat/New Sikalbaha | Southern | CT | 1 | 2017 | Gas | 150 |
Meghnaghat New Site | Dhaka | CC | 1 | 2018 | Gas | 700 |
Plant (Com = Committed) | Region | Unit Type | Unit # | Actual Year of Operation | Fuel | Net Capac, MW |
Madanhat/New Sikalbaha | Southern | CT | 2 | 2018 | Gas | 150 |
Saidpur | Northern | CT | 1 | 2018 | Gas | 150 |
Mawa | Dhaka | CC | 1 | 2019 | Gas | 700 |
Fenchuganj | Central | CT | 1 | 2019 | Gas | 150 |
Mymensingh New Site | Central | CT | 2 | 2019 | Gas | 150 |
Feni | Southern | CT | 1 | 2019 | Gas | 150 |
Meghnaghat New Site | Dhaka | CC | 2 | 2020 | Gas | 700 |
Madanhat/New Sikalbaha | Southern | CC | 1 | 2020 | Gas | 700 |
Amibazar/Dhaka West | Dhaka | CT | 2 | 2021 | Gas | 150 |
Mawa | Dhaka | CC | 2 | 2021 | Gas | 700 |
Baghabari | Northern | CT | 1 | 2021 | Gas | 150 |
Barisal | Western | CT | 1 | 2021 | Gas | 150 |
Mawa | Dhaka | CC | 3 | 2022 | Gas | 700 |
Madanhat/New Sikalbaha | Southern | CC | 2 | 2022 | Gas | 700 |
Mawa | Dhaka | CC | 4 | 2023 | Gas | 700 |
Khulna New | Western | CC | 1 | 2023 | Gas | 700 |
Khulna New | Western | CT | 1 | 2023 | Gas | 150 |
Ghorasal | Dhaka | CC | 1 | 2024 | Gas | 700 |
Khulna New | Western | CC | 2 | 2024 | Gas | 700 |
Ashuganj | Dhaka | CC | 1 | 2025 | Gas | 700 |
Fenchuganj | Central | CT | 2 | 2025 | Gas | 150 |
Baghabari | Northern | CT | 2 | 2025 | Gas | 150 |
Rangpur | Northern | CT | 1 | 2025 | Gas | 150 |
Bheramara | Western | CT | 1 | 2025 | Gas | 150 |
Total | | | | | | 20,495 |
1.3.5 Generation Expansion Plan (Section 6)
Our overall objective is to develop a least-cost generation expansion plan for the Bangladeshi power system covering the period 2005-2025. The generation expansion plan identifies the size, technology, fuel, and timing for new generating plants. The process of establishing the plan also permits development of large data sets tabulating the costs, fuel use, reliability, and other factors useful in analyzing issues relevant to decision-making.
Several sub-objectives support that overall objective.
- Establish a base case scenario and corresponding generation expansion plan for mid-range or most likely conditions. This provides a reference point for further analysis.
- Evaluate the impact of changes to base case conditions through development of a set of scenarios. If the resource plans for different cases are similar over a range of conditions in input variables such as demand or fuel price, one can have more confidence in starting with implementation of the base case plan. Changing conditions are less likely to mandate changes to the resource plan that would be too disruptive or costly. Furthermore, evaluating scenarios can also demonstrate the costs or benefits and other consequences of making choices in areas where choices are possible, such as fuel type.
- Determine the value of the energy and capacity supplied by generation options to establish an upper limit on what should be paid to construct and operate them. Where the cost, in particular the construction or fuel cost, of a generation option is especially uncertain, it can be extremely useful to determine the value of the
energy and capacity provided by that option. This is accomplished by calculating the cost of replacing those services, which often can be easily done with the analytical tools used for generation planning. This replacement cost is an upper limit on what should be paid (using least-cost principles) to construct and operate the option. The option need not be a power plant – it could be a DSM program, a capacity and energy purchase, a program to improve existing plants, or others.
The general approach is to insert a plant with similar operating characteristics to the option of interest, but with zero capital, fuel, and O&M costs. Compared to the Base Case, this “free” plant will reduce the need for other new units and will reduce capital, fuel, and O&M costs. The amount of the overall cost reduction is the potential value to the system of the option of interest.
We used two complementary kinds of analysis. The starting point for both of them is comprehensive data about Bangladesh’s power system, including the costs and performance of existing and potential new generating units.
The first part of the analysis used screening curves. The method consists of developing generation cost curves that show the type of unit that is most economical at each capacity factor. Screening curves plot the annual total cost of electricity from a unit over the range of capacity factors from 0% to 100%. One type might be most economical at low capacity factors and would be most suitable for peaking duty. Another might be most economical at high capacity factors and would be most suitable for base load duty. The curves provide a convenient initial comparison of different technology and fuel types.
The second part of the analysis used a sophisticated computer program for power system optimization and simulation to develop optimal generation expansion plans for the Bangladeshi power system. Based on extensive inputs about the existing system, future conditions, and characteristics of candidate technologies for generation expansion (new power plants), the optimization program provides answers on the type, size, and timing of plant additions during the planning period. The goal is to install new generation capacity on an economic basis while maintaining system reliability. The difference from the screening curve analysis is that in this analysis the technology type and capacity decisions also depend on the size of the units relative to the existing power system, how the new units are to be operated among the existing generating units, the optimal level of reliability, and on the future load growth.
We developed the Base Case scenario and corresponding generation expansion plan based on mid-range or most likely conditions to provide a reference point for further analysis. We defined several scenarios to investigate the impact of changes to Base Case conditions through the use of production simulation and system optimization.
- High and low demand growth rates.
- Limited gas availability, leading to a need for plants using coal fuel.
- High and low cost of energy not served.
- High and low discount rates.
- No application of the LOLP criterion in resource planning.
We defined two scenarios to estimate the value of potential new resources.
- A new 500 MW base load power unit.
- A new 1,000 MW interconnection to neighboring countries providing energy equivalent to a 50% capacity factor.
In addition, we studied some scenarios using screening analysis exclusively or in part.
- Unit size of different technologies.
- Peaking and base load technologies
- Nuclear and diesel units.
- Use of natural gas in steam units.
- High and low gas prices.
- Breakeven price of coal.
- Unit cost of energy not served.
1.3.5.2 Screening Analysis Results
The key screening analysis results are shown in 31-2. The least cost curve consists of ENS for capacity factors from zero to about 2% , the 150 MW SCGT from 2% to about 15%, and the 700 MW CC above 15%. For the first few years we assume the 700 MW CC will not be used. During that time the least cost curve includes the 150 MW SCGT from 2% to about 25% capacity factors and the 450 MW CC above 25%. The steam technologies and the diesel unit are not very close to competitive at any capacity factor.
Figure 1-3 All Technologies and Least Cost Curve
1.3.5.3 Base Case Results
Table 1-8 shows Base Case production simulation and system optimization results for resource additions and reliability statistics. Table 1-9 shows the corresponding costs. Figure 1-4 shows the fuel requirements.
Table 1-8 Base Case Unit Additions and System Reliability Indices
| | Unit Additions, Number of Units | | System Reliabilty Indices |
Year | Peak Load, MW | 700 MW CC | 450 MW CC | 150 MW SCGT | Installed Capacity, MW | LOLP, % | ENS, GWH | Reserve Margin, % |
2005 | 4,308 | 0 | 0 | 0 | 4,458 | 8.138 | 180.8 | 3% |
2006 | 4,693 | 0 | 0 | 0 | 4,683 | 10.884 | 320.9 | 0% |
2007 | 5,112 | 0 | 0 | 0 | 5,425 | 6.350 | 137.5 | 6% |
2008 | 5,569 | 0 | 0 | 2 | 6,002 | 5.135 | 108.3 | 8% |
2009 | 6,066 | 0 | 1 | 0 | 7,313 | 0.845 | 8.9 | 21% |
2010 | 6,608 | 0 | 2 | 0 | 7,986 | 0.750 | 8.2 | 21% |
2011 | 7,148 | 0 | 1 | 1 | 8,586 | 0.797 | 9.0 | 20% |
2012 | 7,732 | 0 | 2 | 0 | 9,449 | 0.490 | 5.1 | 22% |
2013 | 8,364 | 0 | 1 | 1 | 9,979 | 0.834 | 10.1 | 19% |
2014 | 9,047 | 0 | 2 | 0 | 10,879 | 0.654 | 7.4 | 20% |
2015 | 9,786 | 0 | 2 | 1 | 11,579 | 0.937 | 12.6 | 18% |
2016 | 10,512 | 0 | 2 | 0 | 12,479 | 0.848 | 11.2 | 19% |
2017 | 11,291 | 0 | 0 | 5 | 13,229 | 0.997 | 13.5 | 17% |
2018 | 12,128 | 1 | 0 | 2 | 14,229 | 0.912 | 12.2 | 17% |
2019 | 13,027 | 1 | 0 | 3 | 15,243 | 0.880 | 11.9 | 17% |
2020 | 13,993 | 2 | 0 | 0 | 16,643 | 0.578 | 6.7 | 19% |
2021 | 14,924 | 1 | 0 | 3 | 17,455 | 0.816 | 11.2 | 17% |
2022 | 15,917 | 2 | 0 | 0 | 18,526 | 0.949 | 15.6 | 16% |
2023 | 16,977 | 2 | 0 | 1 | 19,867 | 0.811 | 12.5 | 17% |
2024 | 18,107 | 2 | 0 | 0 | 21,070 | 0.923 | 15.9 | 16% |
2025 | 19,312 | 1 | 0 | 4 | 22,370 | 0.950 | 16.1 | 16% |
| Total | 12 | 13 | 23 | 48 | | | |
| Total MW | 8,400 | 5,850 | 3,450 | 17,700 | | | |
| Percent | 47% | 33% | 19% | ^ Total Units and MW Added | |
Table 1-9 Base Case System Costs
| All Costs in Millions of 2005 US$ |
Year | Operating Costs | Capital Costs | Total Direct Costs | ENS Costs | Total Costs |
Fixed & Variable O&M | Fuel |
2005 | 124 | 702 | 0 | 826 | 78 | 904 |
2006 | 138 | 738 | 63 | 938 | 138 | 1,076 |
2007 | 157 | 811 | 305 | 1,273 | 59 | 1,332 |
2008 | 161 | 867 | 478 | 1,506 | 47 | 1,552 |
2009 | 180 | 924 | 467 | 1,570 | 4 | 1,574 |
2010 | 185 | 964 | 518 | 1,667 | 4 | 1,670 |
2011 | 193 | 1,036 | 467 | 1,696 | 4 | 1,700 |
2012 | 202 | 1,101 | 546 | 1,849 | 2 | 1,851 |
2013 | 210 | 1,177 | 636 | 2,023 | 4 | 2,028 |
2014 | 220 | 1,258 | 604 | 2,082 | 3 | 2,085 |
2015 | 232 | 1,347 | 349 | 1,928 | 5 | 1,933 |
2016 | 243 | 1,434 | 494 | 2,170 | 5 | 2,175 |
2017 | 256 | 1,569 | 576 | 2,401 | 6 | 2,407 |
2018 | 269 | 1,678 | 750 | 2,697 | 5 | 2,703 |
2019 | 273 | 1,583 | 645 | 2,501 | 5 | 2,506 |
2020 | 288 | 1,683 | 787 | 2,758 | 3 | 2,761 |
2021 | 294 | 1,767 | 832 | 2,893 | 5 | 2,898 |
2022 | 304 | 1,862 | 819 | 2,984 | 7 | 2,991 |
2023 | 316 | 1,968 | 589 | 2,874 | 5 | 2,879 |
2024 | 328 | 2,080 | 309 | 2,717 | 7 | 2,724 |
2025 | 346 | 2,210 | 0 | 2,556 | 7 | 2,563 |
Total | 4,916 | 28,758 | 10,236 | 43,910 | 402 | 44,313 |
Total NPV | 1,469 | 8,268 | 3,042 | 12,779 | 270 | 13,050 |
Figure 1-4 Base Case Fuel Use
1.3.5.4 Selected Results from High Demand, Low Demand, and Limited Gas Scenarios
Figure 1-5 compares total costs and natural gas use in the Base Case, High Demand, and Low Demand scenarios. The results are as expected. Costs and gas use are proportional to demand.
Figure 1-6 compares the total costs for the Base Case and Limited Gas scenarios. The coal plants in the Limited Gas scenario have higher capital and lower operating costs than the combined cycles in the Base Case. The net impact is the cost of power from the coal plants is higher than for the combined cycles. The capital costs are all incurred before operation, so in the Limited Gas scenario the total costs far exceed those in the Base Case while the plants are being built. As more coal plants become operational, their operating cost advantage brings the total cost back about equal to the Base Case by the end of the study period.
Figure 1-7 shows fuel use in the Limited Gas scenario and compares natural gas use to that in the Base Case. As one would expect, gas use falls off in the Limited Gas scenario, while the use of coal increases dramatically.
Figure 1-5 Comparison of Costs and Natural Gas Use for Demand Growth Cases
Figure 1-6 Comparison of Total Costs, Base Case vs. Limited Gas Case
Figure 1-7 Fuel Use in Limited Gas Case vs. Base Case Natural Gas Use
Analysis of Base Case and the other scenarios we studied produced the following conclusions:
Base Case
- The optimal mix of new resources is fueled by natural gas, with about 20% of new MW coming from SCGT units and 80% from CC units.
- Reliability is low in 2005 – 2007 because there is not enough time to install any units other than the committed units under way.
- Total costs, including the operating costs of the existing system and committed units, and all costs of new units, are about $1.5 billion in 2008 and increase to as much as $3 billion in later years.
- Fuel costs amount to more than 60% of total costs.
- Natural gas requirements grow from 225 BSCF in 2005 to over 700 BCSF in later years.
- The optimal mix of new resources does not change significantly.
- Costs and fuel requirements follow the same trend as demand.
- The optimal mix of new resources includes steam plants fueled by both domestic and imported coal when natural gas is assumed to be unavailable.
- Costs increase significantly when natural gas is not available.
High and Low Demand Scenarios
Limited Gas
- Natural gas requirements fall, offset by large increases in the use of both domestic and imported coal.
- High and low discount rates have very little effect on the optimal resource plan, costs, or fuel requirements.
- When the LOLP criterion is applied, high and low cost of ENS have very little effect on the optimal resource plan, costs, or fuel requirements.
- When the LOLP criterion is not applied, the optimal resource plan changes.
High and Low Discount Rates
High and Low Cost of ENS
No Application of LOLP Criterion
− Fewer MW of new units are added, and SCGT form a larger percentage of the mix.
− The optimal resource plans all have lower reserve margins and higher LOLP and ENS than the Base Case.
− The lower the unit cost of ENS, the higher the LOLP and amounts of ENS, and the lower the reserve margin.
- The application of the LOLP criterion leads to higher overall system costs, given the values for the other parameters we are using.
- The application of the LOLP criterion has little effect on fuel requirements.
Value Scenarios
To calculate the value of a potential new resource, we insert it in the resource plan with zero capital, fuel, and O&M costs. We then calculate the costs of building and operating the generation system when this zero-cost plant is included. Compared to the Base Case, this “free” plant will reduce the need for other new units and will reduce capital, fuel, and O&M costs. The amount of the overall cost reduction is the potential value to the system of the option of interest.
New 500 MW Generating Unit
- The new unit has value close to the costs associated with the unit it replaces, a 450 MW gas fueled CC operating at about 75% capacity factor.
- The new unit’s operating value is $24/MWH. Its total value is $38/MWH assuming operation at about 75% capacity factor.
New 1,000 MW Interconnection
- The new interconnection has value close to the costs associated with the units it replaces, two 450 MW gas fueled CC operating at about 50% capacity factor.
- The new interconnection’s operating value is $24/MWH. Its total value is
$45/MWH assuming operation at about 50% capacity factor.
1.3.6 Transmission Expansion Plan (Section 7)
In the process of conducting the transmission studies for the Master Plan the first step consisted of establishing reliability criteria to be used in the study. The criteria were developed and presented to PGCB and Power Cell planning staff for discussion.
The horizon year planning approach was used to conduct the transmission studies. Here, following definition of the Base Case transmission configuration, which for this study is 2010, the subsequent study involved development of transmission alternatives for the horizon year 2025. The horizon year offers the best opportunities for optimization and affects the development of the transmission system in the intermediate years.
Initially load flow and contingency analysis were conducted to develop the transmission plan in the horizon year. Short circuit and stability analysis were conducted to determine the fault current levels and the dynamic response of the 2025 plan.
Subsequently, we conducted staging, which is the process of determining when each of the components of the horizon year plan is needed to meet planning criteria. This identified the staging of transmission projects in five year increments starting from 2010.
Finally, the robustness of the Master Plan was tested via sensitivity studies that looked into alternate dispatches that could impose local stresses on the transmission network. These studies led to the identification of additional transmission projects to address specific conditions in the future, and thus improved the plan’s robustness. A by-product of the studies is the identification of must-run generators that are required in intermediate years to meet reliability criteria.
The Master Plan is presented in a detailed discussion of transmission lines, transformers and shunt compensation included in the plan, in five year increments from 2010, 2015, 2020, and 2025.
1.3.7 Economic and Financial Analysis (Section 8)
The TOR’s component B, Task 3 (vi) requires the consultant to “Assist BPDB, PGCB, and PMU to prepare financial projections up to the year 2025, with emphasis on cash flows for the recommended power system expansion plan, and conduct economic analyses to determine if the proposed development program is justified.”
We note that this differs from the more detailed financial analyses, plans, and projections required by component C, items 4 (iv), (v), and (vi). Those items will be addressed in Phase 2 of this study.
1.3.7.1 Economic Analysis
Our economic analysis demonstrates how the general approach to generation and transmission planning produces proposed projects and a development program that is justified on an economic basis.
The objective of the generation expansion planning process is to develop a least cost plan subject to certain constraints. The least cost plan includes a schedule of additions of different generating plants of specific size, technology, fuel, efficiency, and other parameters. By least
cost we mean that replacing any of these additions with a different plant, or changing the schedule of additions, will increase the overall cost of the plan.
Accordingly, the plan itself is economically justified 1) If no other plan costs less; and 2) If each plant in the plan is economically justified because replacing it with a different plant; or not replacing it at all, increases overall costs.
We demonstrate that both of the above are true for the Base Case plan in the following manner.
- The appropriate base load and peaking technologies according to the screening analysis are included in the plan.
- The production simulation shows that in the long term the new plants operate within their optimal capacity factor range as indicated in the screening analysis.
- The resulting resource plan is stable because the program, through thousands of simulations, has determined that adding or subtracting any resource will increase overall costs.
- Over a range of parameters the system is far more reliable than the current system, which is widely believed to provide less than optimal reliability.
Using our basic assumptions, we conclude that the plan as a whole is a least cost plan, and its individual new projects are also least cost. Therefore we conclude that the proposed generation development program is justified.
We also consider three of the key constraints and other input assumptions upon which the plan is based: demand growth, gas price, and reliability requirements. Our analysis produces the following conclusions.
- In the near term there is no doubt that the system needs more capacity and aggressive action should be taken to achieve that result.
- In all three demand growth scenarios, by 2009 the plans call for at least one 450 MW CC. By 2010 the plans call for at least one 150 MW SCGT and two 450 MW CCs.
- Using higher gas prices has little impact on the least-cost capacity factor range of the gas turbines and combined cycles.
- Using gas prices as low as the current charges from Petrobangla to BPDB does significantly influence the least-cost capacity factor range of the gas turbines and combined cycles.
- In our opinion, using a low gas price in analysis and building primarily SCGTs as a result would be wasteful of a resource that has high long-term value.
- For the unit costs of ENS that we assume, applying an LOLP criterion, such as is done in the Base Case, results in higher costs than when only an economic test is used.
- Two areas worthy of more investigation are to evaluate whether the LOLP criterion should be applied, and to develop a Bangladesh-specific estimate of the most appropriate value for COENS.
- A study to develop joint least cost electric transmission and gas transmission plans should be addressed as a priority matter.
1.3.7.2 Financial Analysis
The financial analysis forecasts the annual expenditures to 2025 required by the master plan program of generation and transmission additions.
The generation and transmission investment requirements over the next twenty years are substantial, averaging US$2.7 billion for generation and US$0.3 billion for transmission every five years. In order to meet the projected electricity demand, the necessary investments will need to be implemented in a timely manner. This will require careful planning and timely resource mobilization, both public and private. Investment requirements over the next five years to June 2010 are estimated at US$3.2 billion for generation and US$0.6 billion for transmission.
The investment plan over the next five years is quite ambitious, requiring US$1.047 billion in GOB budgetary support and funding from internal cash generation of BPDB and PGCB, US$0.582 billion in new funding support from donors, and US$0.808 billion in “new” private sector investment. Based on existing tariffs, operational performance, and DESA’s payment record of its import energy bills from BPDB, investment funding from internal cash generation of BPDB and PGCB will be minimal and, consequently the burden on the central treasury will be substantial. The annual average support from GOB over the next five years for generation and transmission investments (excluding distribution) will be in the region of US$200 million, compared to the annual average of approximately US$100 million during 2003/04 and 2002/03. An increase of 100% in annual GOB support may not be sustainable in the medium to long-term, and donors may be reluctant to provide further support unless concrete steps are taken to achieve and sustain the financial viability of the power sector. This implies further efficiency improvements and a phased tariff adjustment implementation plan that will lead to cost reflective tariffs over the next two to three years. Ultimately, electricity tariffs should be adequate for the power utilities to meet at least 20% of their investment requirements from internal cash generation.
The on-going and committed generation and transmission projects of BPDB and PGCB will require increasingly larger capital contributions (loan and equity) from GOB in the coming two to three years. The projected capital injection from GOB will grow from US$125 million in 2005/06 to US$220 million in 2006/07 and US$292 million in 2007/08; such burdens on the central treasury may be unsustainable. In addition, funding of foreign currency costs have yet to be secured from external lenders for some of these projects, and appraisal and financial closures on some of the “agreed in principle” projects, such as the ADB and WB funded 3*120MW peaking plants at Siddhirganj, may take longer than envisaged. For these reasons, there may be slippages in the projected commissioning dates of the on-going and committed new generating plants, leading to capacity shortages in the short to medium-term. This is a cause for concern and we recommend that a realistic investment plan concerning these generating plants is drawn up by BPDB, in consultation with the Government and donors. In
parallel, a realistic energy supply/demand balance needs to be prepared for the next two to three years.
1.4 RECOMMENDATIONS
Our general recommendation is to follow the Base Case as the framework for planning and future development of the power system. This least-cost plan is based on our current view of the most likely values for the key input parameters.
We recommend below that the PSMP should be updated on a yearly basis. In other words, a new Base Case would replace the one contained in this document. For example, if no new gas reserves were found in a timely manner, a scenario similar to the Limited Gas scenario of this report would probably become the new Base Case.
We offer other recommendations in four general areas.
1.4.1 Eliminate Load Shedding
The most urgent need is to expand the generation and transmission systems to eliminate the routine load shedding that has prevailed for the last ten years and is forecast to continue for the next few years at least. Five recommendations address that critical issue:
- Construct and place in operation the committed generation units as quickly as possible.
- Construct and place in operation the committed transmission projects as quickly as possible.
- Secure financing for committed projects whose financing is not certain.
- Initiate the process leading to implementation of two new 150 MW SCGTs (planned for Sikalbaha and Bogra) by 2008 and at least one 450 MW CC (planned for Meghnaghat) by 2009 and two such units by 2010 (the second one planned for Sikalbaha).
- Initiate the process leading to implementation the transmission plan for 2010.
1.4.2 Near-Term Strategy
The recommended near-term strategy includes the following elements:
- Use natural gas fueled SCGT for peaking duty and CC for mid-range and base load service as the primary, and possibly exclusive, resource plan additions as long as the resource base indicates sufficient gas to supply them.
- BPDB and PGCB should develop a realistic investment plan concerning planned generation and transmission, in consultation with the GOB and donors.
- Use the Base Case demand forecast for planning purposes until a new demand forecast is developed.
- Use a liquid fuel based natural gas price for purposes of resource plan analysis. This should not be used for pricing gas sales to generators or setting prices to IPPs unless that is decided as a policy of the GOB.
- Undertake a study of the joint least cost development of the natural gas transmission system, the electric transmission system, and the electric generation system.
1.4.3 Assure Natural Gas Availability
The generation expansion plan is based nearly entirely on the premise that natural gas will continue to be available. Assuring that this premise continues to apply is critical.
- Monitor the status of natural gas reserves and deliverability as part of routine electricity planning activities.
- Take the needs of the power sector into account in planning the development of gas fields and transport systems.
1.4.4 Reduce Uncertainty
Many near-term, mostly low-cost study activities will reduce risks and uncertainties, and improve both near- and long-term planning results.
- Update the PSMP on a yearly basis. BPDB and PGCB have the updated planning models and experience to do this with little or no assistance from consultants.
− Some training of BPDB and PGCB in the use of their new models and some of their special applications would be beneficial, but is not covered as part of the current PSMP project.
- Consider using higher emergency ratings than normal rating for post-contingency conditions for transformer and transmission line loadings.
- Monitor the cost and performance worldwide of larger units such as the 700 MW CC in order to gain confidence that they would be suitable for Bangladesh.
- Evaluate the need for application of the LOLP criterion.
- Develop a Bangladesh-specific estimate of the most appropriate value for unit cost of ENS.
- Develop an information base for the cost of domestic and imported coal in Bangladesh.
- Develop a better data base to support dynamic analysis of the transmission system, including data on IPP generators.
- Investigate the feasibility of alternative right-of-ways to support transmission system development.
- Encourage broader use of power from private generators such as co-generators and self-generators.
− Establish procedures to guarantee ability to safely interconnect and for backup power from the grid.
− Rationalize pricing.
- Conduct more detailed analysis of the value of new resources to support decision- making in specific cases.
Section 2 Existing Power System
2.1 OVERVIEW OF EXISTING POWER SYSTEM
Three key features have shaped Bangladesh’s power system historically. First is the relative concentration of demand close to Dhaka municipality, and to a much lesser extent close to Chittagong and Khulna. Second is the source in the fields of the eastern part of the country of the natural gas that now fuels most of the power plants. Third is the isolating effect of the rivers that divide the east and west, making transport of fuel or electricity from east to west relatively difficult and expensive.
A key non-geographic factor is that generating capacity has continually fallen short of what is needed to meet demand. Significant load shedding due to lack of generation occurred in each of the historical years reviewed. In fiscal 2004, estimated maximum load shedding was 694 MW, with load shedding at time of peak of 461 MW. This is consistent with the experience of the last ten years. In 2004, estimated demand after adding estimated load shedding to measured demand was 20,283 GWH and 3,952 MW. De-rated installed net generating capacity was 4,120 MW.
In 2003 demand in the Dhaka region was 47% of national demand. The Southern Region, including Chittagong, had 20%. The Western Region, including Khulna, had 12%. The Central Region had 8% and the Northern Region 12%.
Placing power plants close to Dhaka minimized transmission costs and losses, and helped maintain voltages. Dhaka is also relatively accessible from the eastern gas fields. Thus in general terms the generation system today consists of multiple plants with most of national capacity near Dhaka, with smaller plants near the gas fields or other load centers. Some plants rely on heavy oil or distillate. The highest voltage transmission system consists of a 230 KV loop around Dhaka with radial extension to the other regions. The 132 KV system initially extended radially from Dhaka to the other regions, but now includes loops ringing Dhaka and Chittagong, and larger loops in the Southern, Western, and Northern regions.
In April 2005 PGCB prepared a map of the existing system that we include here as Figure 2-1.
Section 4 addresses both the existing and possible future fuel supply systems for the power plants, so we do not address the existing fuel supply system in this section.
Figure 2-1 Map of Existing System
2.2 EXISTING AND COMMITTED GENERATION PROJECTS
The generation planning process starts from the existing system. Considering load growth, retirements of existing plants, and other factors, the process determines the new generation that is needed in addition to the existing generation. Thus a tabulation of generating plants that comprise the existing system is needed.
We also include “committed” plants in the planning process. These are plants that are not yet operational, but that are far enough along in the process of approving, financing, and building that it is highly likely that it will be built and become operational. In other words, these are plants that are not subject to being displaced by new units that the generation planning process may identify.
Table 2-1 summarizes the generation totals by region. Table 2-1 shows that Dhaka has over 61% of the national total existing generation capacity, and on average has larger units than all other regions except the Southern. Its average unit size is larger than Southern when one accounts for the fact that for simplicity we have treated the Southern five-unit hydro plant as a single unit. Dhaka’s share of national capacity will fall as the committed units come on line, and later as the trend continues to place new plants so that the regional demand and generation are more in balance.
Table 2-1 Generation Capacity by Region
Region | Dhaka | Central | Southern | Northern | Western | National |
Number of Existing Units June 2004 | 23 | 11 | 4 | 4 | 16 | 58 |
Existing Generation June 2004, Derated Net MW | 2,506 | 345 | 605 | 260 | 404 | 4,120 |
% of National Total MW | 61% | 8% | 15% | 6% | 10% | 100% |
MW/Unit | 109 | 31 | 151 | 65 | 25 | 71 |
Number of Committed Units | 8 | 3 | 2 | 5 | 1 | 19 |
Committed Generation, Derated Net MW | 1,342 | 257 | 199 | 850 | 197 | 2,845 |
% of National Total MW | 47% | 9% | 7% | 30% | 7% | 100% |
Number of Existing and Committed Units | 31 | 14 | 6 | 9 | 17 | 77 |
Total Existing and Committed, Derated Net MW | 3,848 | 602 | 804 | 1,110 | 601 | 6,965 |
% of National Total MW | 55% | 9% | 12% | 16% | 9% | 100% |
Table 2-2 summarizes the primary energy resources for the generation. Natural gas is the predominant fuel and will become even more dominant over the next few years.
Table 2-2 Primary Energy Resources for Existing and Committed Capacity
| PRIMARY ENERGY INPUT | |
| FUELS | | |
| NaturalGas | DomesticCoal | ImportedCoal | Diesel | HeavyFuelOil | Hydro | TOTAL |
Total National Existing Capacity, MW | 3,486 | 0 | 0 | 158 | 246 | 230 | 4,120 |
% of National Total | 85% | 0% | 0% | 4% | 6% | 6% | 100% |
Total National Committed Capacity, MW | 2,515 | 230 | 0 | 0 | 0 | 100 | 2,845 |
% of National Total | 88% | 8% | 0% | 0% | 0% | 4% | 100% |
TOTAL NATIONAL EXISTING AND COMMITTED CAPACITY, MW | 6,002 | 230 | 0 | 158 | 246 | 330 | 6,966 |
% of National Total | 86% | 3% | 0% | 2% | 4% | 5% | 100% |
Table 2-3 presents the detailed cost and performance parameters for the existing and committed projects in Bangladesh. The source of the data was BPDB records, enhanced through discussions with BPDB management and staff. The terms used in Table 2-3 have the following meanings:
- Type: CC = combined cycle, SCGT = simple cycle gas (combustion) turbine, ST
= steam turbine, DIE = diesel engine, HY = hydro
- # of Units June 04: The number of units of that type and size at the site that existed as of June 2004
- Min Load, MW: The lowest load, in MW, at which the unit can operate reliably
- Unit Net Capacity, MW: The maximum net power that can reliably be delivered into the grid from that unit. This figure is referred to the high side of the main power plant transformer, in other words it represents the power level after subtracting plant auxiliary and main transformer losses from generator output.
- Unit Set Net Cap, MW: Unit net capacity for that set of units of the same type and size = the net capacity of each unit times the number of units
- Heat Rates, KCAL/KWH: The amount of fuel necessary to produce one KWH of generation. Min Load refers to the amount of fuel needed when the unit is operating at minimum load. Incremental refers to the additional fuel needed for one additional KWH when the unit is operating at power levels above minimum. At other power levels the actual heat rate is determined by the formula:
HRX = ((HRMin * Min Load) + (HRIncr * (OutputX – Min Load))) / OutputX HRX = Heat rate at power output level X above Min Load
HRMin = Heat rate at Min Load HRIncr = Incremental heat rate
OutputX = Output in MW at power output level above Min Load
- Fuel Costs, US Cents Per 10^6 KCAL: The cost in US cents per million KCAL of the fuel used for each plant. The cost represents the levelized cost based on forecast fuel prices over the study period 2005 – 2025. Domestic refers to costs for fuels produced in Bangladesh. Foreign refers to fuels that are imported from foreign sources.
- Fuel Type: Gas = natural gas, Dom Coal = coal mined in Bangladesh, Imp Coal
= imported coal’ HSDIE = high sulfur diesel oil, HSFO = high sulfur fuel oil, Hydro = hydro plant – does not use fuel
- FOR,%: The unit’s forced outage rate, in %
- Days/yr Sched Maint: The number of days per year that the unit must be out of service due to scheduled maintenance
- Maint Class, MW: The unit’s maintenance class, which the planning program uses in scheduling maintenance for the different units
- O&M Costs: Used to calculate the cost of operating and maintaining the unit. Fixed O&M, $/KW-Mo is the cost of O&M that is independent of the amount of energy generated. For example, this would include the personnel costs of the plant that apply whether or not the unit operates. The cost each month is determined by multiplying the unit’s net capacity by the listed value. Var,
$/MWH is the variable cost of O&M that depends on the energy output of the unit. For example, this would include chemicals and makeup lubricating oil. Fuel is not included in this category. The cost for a given time period is determined by multiplying the unit’s net energy output in MWH by the listed value.
- Notes: The notes section at the bottom of the table explains some entries.
- Operating Dates: The fiscal year of initial commercial operation for all committed units. Not given for existing units.
- Retirement Years: The fiscal year when a unit is removed from service. Not shown for units that do not retire during the study period.
The retirement years shown in Table 2-3 were based on the unit typical lifetimes shown below. However, the specific forecast retirement dates for the existing units also take into account the present condition of the units, maintenance costs, fuel and fuel cost, and efficiency. In practice each power plant retirement decision should be made at an appropriate time, such as when a major maintenance expense is being considered. The decision should be based on a technical/economic analysis that considers the above and other relevant factors such as the then-current balance of demand and generation.
- Steam turbine based units: 30 years
- Combined cycles based on combustion turbines: 25 years
- Simple cycle combustion turbines: 20 years
Many of the columns of Table 2-3 provide information necessary only for fuel-burning units. Bangladesh has only a single significant hydro plant. Karnafuli’s existing five turbines (two of 40 MW and three of 50 MW) provide a maximum of 230 MW of generating capacity.
Two additional 50 MW units are committed, bringing total maximum capacity to 330 MW.
Determining the available capacities of hydro units and plants is more complicated than for fuel-burning plants. The maximum instantaneous capacity depends on the water levels in the reservoir and the afterbay. However, that maximum capacity is not available all the time because typically reservoir inflows do not provide enough water to operate the units around the clock. Furthermore, typically operators manage reservoirs to serve several needs, such as agriculture, flood control, and recreation as well as power production. For power production, operators manage reservoirs to maximize the value of the power generated by delivering as much as possible it into peak load periods. With these considerations and this approach, a hydro plant may have effective capacity less than installed capacity. For example, running a plant at full capacity during the entire peak period today may mean that reservoir water levels are lower and less water is available for discharge during tomorrow’s peak periods. Both these factors reduce effective capacity. Generation planning programs take this into account by modeling the plants by season, with each season potentially having a different effective maximum capacity and total energy generation. Karnafuli was modeled in this manner.
Hydro units typically have low forced outage rates. Because the effective capacity is sometimes less than the maximum capacity, the impact of forced outages is less. If one unit is forced out, the remaining actual capacity may still exceed the effective capacity used in the program.
BPDB has undertaken a project under the title ‘Power Plant Rehabilitation Project (Phase 2) Revised’ which is currently under implementation.
The scope of this project is designed to improve improve operational performance including efficiency improvement from a number of ‘maintenance-overdue’ units, through rehabilitation of 19 of them, in 8 power stations. The work included a local feasibility study, supply of mechanical spares, electrical spares, new equipment and materials for the affected power plant generating units and electrical substations. The project cost was estimated at US$ 26.5 million in foreign exchange, plus TK 679 million in local currency.
Table 2-4 lists the units to be rehabilitated and their status. The project was scheduled to be complete by the year 2006.
Table 2-5 summarizes the status of the committed projects that total 2,845 MW. The generation expansion plan is based on achieving the capacities and operating dates (fiscal year) of all those units.
Delays in competing the rehabilitation projects, or more significantly the committed projects, will reduce the amount of generation available to meet load in the first few years of the planning period. Thus it is especially important to complete these projects, because in many cases there is not enough time to replace their generation with new plants. The current status indicates that most of the projects are progressing towards completion on or close to schedule. However, in some cases funding has not been completed. Many of the projects rely on GOB funding, for which there may be competing needs.
Table 2-3 Existing and Committed Generation
| | | | | | Heat Rates, KCAL/KWH | Fuel Costs, US Cents Per 10^6 KCAL | | | | | O&M Costs | | | |
| Type | # of Units June 04 | Min Load, MW | Unit Derated Net Capacity, MW | Unit Set Derated Net Cap, MW | Min Load | Incremental | Domestic | Foreign | Fuel Type | FOR, % | Days/yr Sched Maint | Maint Class, MW | Fixed, $/KW-Mo | Var, $/MWH | Notes | Operating Dates | Retirement Years |
DHAKA REGION | | | | | | | | | | | | | | | | | | |
Regional Existing June 2004 | | | | | | | | | | | | | | | | | |
ASHUGANJ | ST | 2 | 30 | 60 | 120 | 3,500 | 2,232 | 1,265 | 0 | Gas | 7 | 60 | 60 | 2.00 | 2.00 | | | 2008 |
ASHUGANJ | ST | 3 | 70 | 141 | 423 | 2,953 | 1,882 | 1,265 | 0 | Gas | 4 | 60 | 150 | 2.00 | 2.00 | | | 2021, '22, '23 |
ASHUGANJ | CC | 1 | 30 | 60 | 60 | 3,483 | 2,137 | 1,265 | 0 | Gas | 15 | 45 | 60 | 1.25 | 1.30 | | | 2010 |
ASHUGANJ | SCGT | 1 | 40 | 40 | 40 | 4,258 | 4,258 | 1,265 | 0 | Gas | 15 | 30 | 60 | 1.00 | 2.50 | | | 2010 |
GORASAL UNIT 2 | ST | 1 | 26 | 37 | 37 | 3,232 | 2,189 | 1,265 | 0 | Gas | 10 | 60 | 60 | 2.00 | 2.00 | | | 2012 |
GORASAL | ST | 4 | 100 | 197 | 788 | 2,947 | 1,884 | 1,265 | 0 | Gas | 8 | 60 | 200 | 2.00 | 2.00 | | | 1 in '21 1 in '24 |
HARIPUR | SCGT | 3 | 30 | 30 | 90 | 3,835 | 3,835 | 1,265 | 0 | Gas | 5 | 30 | 30 | 1.00 | 2.50 | | | 2010 |
IPP HARIPUR BARGE | DIE | 5 | 11 | 22 | 110 | 2,000 | 2,000 | 1,265 | 0 | Gas | 7 | 30 | 30 | 0.00 | 2.52 | | | 2015 |
CDC HARIPUR | CC | 1 | 180 | 360 | 360 | 2,027 | 1,479 | 1,265 | 0 | Gas | 7 | 45 | 400 | 1.42 | 1.10 | | | |
CDC MEGHNAGHAT | CC | 1 | 225 | 450 | 450 | 1,900 | 1,850 | 1,265 | 0 | Gas | 7 | 45 | 500 | 0.54 | 1.03 | | | |
SIDHIRGANJ | ST | 1 | 18 | 28 | 28 | 3,480 | 2,232 | 1,265 | 0 | Gas | 10 | 60 | 30 | 2.00 | 2.00 | | | 2007 |
Total Regnl Existing June 04 | 23 | | | 2,506 | | | | | | | | | | | | | |
Regional Committed | | | | | | | | | | | | | | | | | | |
GORASAL UNIT 1 (Under Maintenance) | ST | 1 | 26 | 37 | 37 | 3,232 | 2,189 | 1,265 | 0 | Gas | 10 | 60 | 60 | 2.00 | 2.00 | 1 | 2005 back on | 2012 |
CDC MEGHNAGHAT NEW UNIT | CC | 1 | 225 | 450 | 450 | 1,900 | 1,850 | 1,265 | 0 | Gas | 7 | 45 | 500 | 0.54 | 1.03 | | 2008 | |
SIDHIRGANJ | ST | 2 | 100 | 197 | 394 | 2,670 | 2,098 | 1,265 | 0 | Gas | 8 | 60 | 200 | 2.00 | 2.00 | | 2005, 2009 | |
SIDHIRGANJ | SCGT | 3 | 60 | 119 | 357 | 3,064 | 2,145 | 1,265 | 0 | Gas | 7 | 30 | 150 | 1.00 | 2.50 | | 2007 | |
TONGI | SCGT | 1 | 52 | 104 | 104 | 3,064 | 2,145 | 1,265 | 0 | Gas | 7 | 30 | 150 | 1.00 | 2.50 | | 2005 | |
Total Regional Committed | 8 | | | 1,342 | | | | | | | | | | | | | |
Total Dhaka Region | | 31 | | | 3,848 | | | | | | | | | | | | | |
| | | | | | Heat Rates, KCAL/KWH | Fuel Costs, US Cents Per 10^6 KCAL | | | | | O&M Costs | | | |
| Type | # of Units June 04 | Min Load, MW | Unit Derated Net Capacity, MW | Unit Set Derated Net Cap, MW | Min Load | Incremental | Domestic | Foreign | Fuel Type | FOR, % | Days/yr Sched Maint | Maint Class, MW | Fixed, $/KW-Mo | Var, $/MWH | Notes | Operating Dates | Retirement Years |
CENTRAL REGION | | | | | | | | | | | | | | | | | | |
Regional Existing June 2004 | | | | | | | | | | | | | | | | | |
FENCHUGANJ | CC | 1 | 44 | 88 | 88 | 2,090 | 2,070 | 1,265 | 0 | Gas | 6 | 45 | 100 | 1.25 | 1.30 | | | 2022 |
SHAHJIBAZAR | SCGT | 3 | 10 | 10 | 30 | 7,425 | 7,425 | 1,265 | 0 | Gas | 15 | 30 | 20 | 1.00 | 2.50 | | | 2007 |
SHAHJIBAZAR | SCGT | 2 | 34 | 34 | 68 | 3,062 | 3,062 | 1,265 | 0 | Gas | 10 | 30 | 60 | 1.00 | 2.50 | | | 2023 |
SYLHET | SCGT | 1 | 19 | 19 | 19 | 3,969 | 3,969 | 1,265 | 0 | Gas | 8 | 30 | 20 | 1.00 | 2.50 | | | 2008 |
RPC MYMENSHUNG UNITS | GT | 4 | 18 | 35 | 140 | 3,250 | 3,250 | 1,265 | 0 | Gas | 7 | 30 | 60 | 5.70 | 0.94 | 2 | | 2006 |
Total Regnl Existing June 04 | 11 | | | 345 | | | | | | | | | | | | | |
Regional Committed | | | | | | | | | | | | | | | | | | |
FENCHUGANJ | CC | 1 | 44 | 88 | 88 | 2,090 | 2,070 | 1,265 | 0 | Gas | 6 | 45 | 100 | 1.25 | 1.30 | | 2008 | |
SYLHET | SCGT | 1 | 50 | 99 | 99 | 3,064 | 2,145 | 1,265 | 0 | Gas | 7 | 30 | 100 | 1.00 | 2.50 | | 2007 | |
MYMENSHING RPC | CC | 1 | 105 | 210 | 70 | 2,000 | 1,900 | 1,265 | 0 | Gas | 7 | 45 | 400 | 5.00 | 1.00 | 2 | 2006 | |
Total Regional Committed | 3 | | | 257 | | | | | | | | | | | | | |
Total Central Region | | 14 | | | 602 | | | | | | | | | | | | | |
| | | | | | | | | | | | | | | | | | |
SOUTHERN REGION | | | | | | | | | | | | | | | | | | |
Regional Existing June 2004 | | | | | | | | | | | | | | | | | |
RAUZAN | ST | 2 | 83 | 164 | 328 | 2,990 | 1,911 | 1,265 | 0 | Gas | 6 | 60 | 200 | 2.00 | 2.00 | | | |
SIKALBAHA | ST | 1 | 24 | 47 | 47 | 3,540 | 2,558 | 1,265 | 0 | Gas | 10 | 60 | 60 | 2.00 | 2.00 | | | 2019 |
KARNAFULI HYDRO HY1 | HY | 1 | | 230 | 230 | | | | | Hydro | | | | 2.00 | | 3 | | |
Total Regnl Existing June 04 | 4 | | | 605 | | | | | | | | | | | | | |
Regional Committed | | | | | | | | | | | | | | | | | | |
CHANDPUR | SCGT | 1 | 50 | 99 | 99 | 3,064 | 2,145 | 1,265 | 0 | Gas | 7 | 30 | 100 | 1.00 | 2.50 | | 2007 | |
KARNAFULI HYDRO HY2 | HY | 1 | | 100 | 100 | | | | | Hydro | | | | 2.00 | | 4 | 2009 | |
Total Regional Committed | 2 | | | 199 | | | | | | | | | | | | | |
Total Southern Region | | 6 | | | 804 | | | | | | | | | | | | | |
| | | | | | Heat Rates, KCAL/KWH | Fuel Costs, US Cents Per 10^6 KCAL | | | | | O&M Costs | | | |
| Type | # of Units June 04 | Min Load, MW | Unit Derated Net Capacity, MW | Unit Set Derated Net Cap, MW | Min Load | Incremental | Domestic | Foreign | Fuel Type | FOR, % | Days/yr Sched Maint | Maint Class, MW | Fixed, $/KW-Mo | Var, $/MWH | Notes | Operating Dates | Retirement Years |
NORTHERN REGION | | | | | | | | | | | | | | | | | | |
Regional Existing June 2004 | | | | | | | | | | | | | | | | | |
BAGHABARI | SCGT | 1 | 70 | 70 | 70 | 3,057 | 3,057 | 1,265 | 0 | Gas | 4 | 30 | 100 | 1.00 | 2.50 | | | 2013 |
BAGHABARI | SCGT | 1 | 100 | 100 | 100 | 3,008 | 3,008 | 1,265 | 0 | Gas | 4 | 30 | 100 | 1.00 | 2.50 | | | 2022 |
BAGHABARI BARGE MTD | SCGT | 2 | 22 | 45 | 90 | 1,960 | 1,960 | 1,265 | 0 | Gas | 7 | 30 | 60 | 0.00 | 2.38 | 5 | | 2006 |
Total Regnl Existing June 04 | 4 | | | 260 | | | | | | | | | | | | | |
Regional Committed | | | | | | | | | | | | | | | | | | |
BARAPUKURIA COAL | ST | 2 | 70 | 115 | 230 | 2,751 | 1,872 | 797 | 0 | Dom Coal | 10 | 60 | 150 | 4.58 | 4.00 | | Both units in 2006 | |
BAGHABARI BARGE MTD | CC | 1 | 65 | 130 | 40 | 2,185 | 1,709 | 1,265 | 0 | Gas | 7 | 45 | 150 | 0.00 | 1.76 | 5 | 2006 | 1 in 2015 |
BAGHABARI BARGE MTD | CC | 1 | 65 | 130 | 130 | 2,185 | 1,709 | 1,265 | 0 | Gas | 7 | 45 | 150 | 0.00 | 1.76 | 5 | 2007 | 1 in 2015 |
SIRAGANJ | CC | 1 | 225 | 450 | 450 | 1,900 | 1,875 | 1,265 | 0 | Gas | 7 | 45 | 500 | 0.54 | 1.03 | | 2009 | |
Total Regional Committed | 5 | | | 850 | | | | | | | | | | | | | |
Total Northern Region | | 9 | | | 1,110 | | | | | | | | | | | | | |
| | | | | | Heat Rates, KCAL/KWH | Fuel Costs, US Cents Per 10^6 KCAL | | | | | O&M Costs | | | |
| Type | # of Units June 04 | Min Load, MW | Unit Derated Net Capacity, MW | Unit Set Derated Net Cap, MW | Min Load | Incremental | Domestic | Foreign | Fuel Type | FOR, % | Days/yr Sched Maint | Maint Class, MW | Fixed, $/KW-Mo | Var, $/MWH | Notes | Operating Dates | Retirement Years |
WESTERN REGION | | | | | | | | | | | | | | | | | | |
Total Regnl Existing June 04 | | | | | | | | | | | | | | | | | |
BHERAMARA | SCGT | 3 | 18 | 18 | 54 | 3,772 | 3,772 | 0 | 2,585 | HSDie | 6 | 20 | 20 | 1.00 | 2.50 | | | 2008 |
KHULNA | ST | 1 | 24 | 47 | 47 | 3,540 | 2,558 | 0 | 1,686 | HSFO | 10 | 60 | 60 | 2.00 | 2.00 | | | 2009 |
KHULNA | ST | 1 | 45 | 89 | 89 | 3,437 | 2,487 | 0 | 1,686 | HSFO | 10 | 60 | 100 | 2.00 | 2.00 | | | 2019 |
KHULNA | SCGT | 2 | 16 | 16 | 32 | 3,454 | 3,454 | 0 | 2,585 | HSDie | 5 | 30 | 20 | 1.00 | 2.50 | | | 2008 |
BARISAL, RANGPUR, SAIDPUR | SCGT | 4 | 18 | 18 | 72 | 4,000 | 4,000 | 0 | 2,585 | HSDie | 8 | 30 | 20 | 1.00 | 2.50 | | | 2 in '08 2 in '09 |
KHULNA IPP | SCGT | 5 | 11 | 22 | 110 | 2,205 | 2,205 | 0 | 1,686 | HSFO | 7 | 30 | 100 | 0.00 | 1.92 | | | 2015 |
Regional Existing June 2004 | 16 | | | 404 | | | | | | | | | | | | | |
Regional Committed | | | | | | | | | | | | | | | | | | |
KHULNA ST#2 | ST | 1 | 100 | 197 | 197 | 2,670 | 2,098 | 1,265 | 0 | Gas | 8 | 60 | 200 | 2.00 | 2.00 | | 2009 | |
Total Regional Committed | 1 | | | 197 | | | | | | | | | | | | | |
Total Western Region | | 17 | | | 601 | | | | | | | | | | | | | |
| | | | | | | | | | | | | | | | | | |
Total National Existing | | 58 | | | 4,120 | | | | | | | | | | | | | |
Total National Committed | | 19 | | | 2,845 | | | | | | | | | | | | | |
TOTAL NATIONAL EXISTING AND COMMITTED | 77 | | | 6,965 | | | | | | | | | | | | | |
| | | | | | | | | | | | | | | | | | |
Note 1: Out of service in 2004, return to service in 2005 | | | | | | | | | | | | | | |
Note 2: 1 new 70 MW ST turns 4 existing 35 MW CT "retired" in 2006 into CC. 70 is net MW added. | | | | | | | | |
Note 3: 2x40 MW UNIS + 3x50 MW UNITS, plant annual energy 797 GWH | | | | | | | | | | | |
Note 4: 2x50 MW units added to existing plant, added annual energy 100 GWH | | | | | | | | | | | |
Note 5: 1 new 40 MW ST turns 2 existing 45 MW CT "retired" in 2006 into 130 MW CC in 2006. 40 MW added. A second similar plant comes on line in 2007. The first plant retires in 2015 | | | | | | | |
| | | | | | | | | | | | | | | | | | |
Table 2-4 Rehabilitation Projects
No. | Unit | Region | Status |
1 | Khulna 110 MW Steam | Western | Excitation system & modernization works will start soon |
2 | Khulna 60 MW Steam | Western | Tender evaluation under process for rehab of exhaust system |
| | | |
3 | Khulna 28 Mounted #1 | MW | SCGT | Barge | Western | Not included in current project |
4 | Khulna 28 Mounted #2 | MW | SCGT | Barge | Western | Not included in current project |
| | | |
5 | Bheramara 20 MW SCGT unit #1 | Western | Notification of Award for rehab of some aux systems done |
6 | Bheramara 20 MW SCGT unit #2 | Western | Notification of Award for rehab of some aux systems done |
7 | Bheramara 20 MW SCGT unit #3 | Western | Notification of Award for rehab of some aux systems done |
| | | |
8 | Ghorasal 210 MW Steam unit #3 | Dhaka | Tender invited for purchase of spare parts |
9 | Ghorasal 210 MW Steam unit #4 | Dhaka | Tender invited for purchase of spare parts |
10 | Ghorasal 210 MW Steam unit #5 | Dhaka | Tender invited for purchase of spare parts |
11 | Ghorasal 210 MW Steam unit #6 | Dhaka | 1) Tender invited for gen excitation system modernization and purchase of spares, etc. 2) Tender under evaluation for turnkey installation of motors |
| | | |
12 | Rangpur 20 MW SCGT | Northern | Spare parts purchased and rehab work to start soon |
| | | |
13 | Fenchuganj 90 MW CC | Central | Tender invited for purchase of spare parts (due 16/07/05) |
| | | |
14 | Raozan 210 MW Steam 2nd unit | Southern | Spare parts purchased and rehab work to start soon |
| | | |
15 | Sikalbaha 60 MW Steam | Southern | Notification of Award for overhaul done (12/06/05) |
| | | |
16 | Kaptai 40 MW Hydro unit #1 | Southern | Awaiting Ministry's approval on tender evaluation report for rehab |
17 | Kaptai 40 MW Hydro unit #2 | Southern | Spare parts purchased and rehab works will start soon |
18 | Kaptai 50 MW Hydro unit #4 | Southern | Spare parts purchased and rehab works will start soon |
19 | Kaptai 50 MW Hydro unit #5 | Southern | Spare parts purchased and rehab works will start soon |
Table 2-5 Status of Committed Generation Projects
| Type | # of Cmtd Units | Unit Net Capacity, MW | Unit Set Net Cap, MW | Status | Notes | ScheduledOperating Dates |
DHAKA REGION | | | | | | | |
GORASAL UNIT 1 (Under Maintenance) | ST | 1 | 37 | 37 | Operational | 1 | 2005 back on |
MEGHNAGHAT PHASE 2 IPP | CC | 1 | 450 | 450 | Bid approved by Cabinet Committee on Purchases (CCP) and NOI issued to bidder - schedule may slip by 1 year | | 2008 |
SIDHIRGANJ | ST | 2 | 197 | 394 | 1 unit operational. 2nd unit bids received and evaluated, awaiting CCP approval. Supplier or GOB financing. | | 2005, 2009 |
SIDHIRGANJ | SCGT | 3 | 119 | 357 | ADB financing signed for 2 units. WB financing expected for 3rd unit. | | 2007 for all 3 |
TONGI | SCGT | 1 | 104 | 104 | Operational | | 2005 |
Total Regional Committed | 8 | | 1,342 | | | |
CENTRAL REGION | | | | | | | |
FENCHUGANJ | CC | 1 | 88 | 88 | Bid from Chinese firm Harbin approved by CCP. GOB funding allocated. Con- tract sgned for implementation. | | 2008 |
SYLHET | SCGT | 1 | 99 | 99 | Bid not approved by CCP. Will be re- tendered. GOB funding expected. | | 2007 |
MYMENSHING RPC | ST/CC | 1 | 70 | 70 | Was under construction, but Siemens has left site awating resolution of controversy. | 2 | 2006 |
Total Regional Committed | 3 | | 257 | | | |
SOUTHERN REGION | | | | | | | |
CHANDPUR | SCGT | 1 | 99 | 99 | Received bid, under evaluation. Awaiting formal approval by GOB. | | 2007 |
KARNAFULI HYDRO HY2 | HY | 1 | 100 | 100 | Donor funding once considered. No agreement reached. | 3 | 2009 |
Total Regional Committed | 2 | | 199 | | | |
NORTHERN REGION | | | | | | | |
BARAPUKURIA COAL | ST | 2 | 115 | 230 | Under construction. Expect to maintain schedule. | | 2006, 2006 |
BAGHABARI BARGE MTD IPP | ST/CC | 1 | 40 | 40 | Unit 1 conversion - schedule is OK. | 4 | 2006 |
BAGHABARI BARGE MTD IPP | CC | 1 | 130 | 130 | Unit 2 contract signed, schedule OK. | 4 | 2007 |
SIRAGANJ IPP | CC | 1 | 450 | 450 | To be re-tendered. Schedule is OK. | | 2009 |
Total Regional Committed | 5 | | 850 | | | |
WESTERN REGION | | | | | | | |
KHULNA ST#2 | ST | 1 | 197 | 197 | Received supplier's credit based proposal, now at Ministry. | | 2009 |
Total Regional Committed | 1 | | 197 | | | |
Total National Committed | 19 | | 2,845 | | | |
Note 1: Out of service in 2004, return to service in 2005 | | |
Note 2: 1 new 70 MW ST turns 4 existing 35 MW CT "retired" in 2006 into CC. 70 is net MW added. |
Note 3: 2x50 MW units added to existing plant, added annual energy 100 GWH | | |
Note 4: 1 new 40 MW ST turns 2 existing 45 MW CT "retired" in 2006 into 130 MW CC in 2006. 40 MW added. A second similar plant comes on line in 2007. | |
2.3 EXISTING TRANSMISSION SYSTEM AND COMMITTED PROJECTS
2.3.1 Existing Transmission System
The existing Bangladesh transmission system is shown in Figure 2-1 and incorporates two main voltage levels, 230 KV and 132 KV. Figure 2-1 includes existing, under construction, and planned facilities. Dashed lines correspond to planned facilities, dotted lines to facilities under construction, and solid lines to existing transmission facilities.
Around Dhaka a 230 KV ring constitutes the bulk power system to transfer power from the generation centers in the eastern part of the city to the load centers throughout the city. In addition there is a 132 KV system that carries the power flow through the 132 KV substations to the loads.
Currently, there is a 230 KV transmission line that ties the eastern and western part of the country. This line goes from the Ghorasal substation in the northeast part of Dhaka to Ishurdi, a substation located in the western part of Bangladesh. There is, under construction, a second 230 KV line that will interconnect the east and west portions of the country contributing then to a significant improvement of the reliability of PGCB’s transmission system. This line will go through the Jamuna Bridge from the Ashuganj substation in the eastern part of the country to the Sirajganj substation in the west.
A 230 KV line from the southeast part of Dhaka goes through the 230 KV Comilla North substation to Hathazari 230 KV substation located in the Southern region, in the Chittagong area. A 230 KV line from the 230 KV Comilla North substation extends to the Ashuganj 230 KV substation, interconnecting the Central and Southern regions of Bangladesh.
Another 230 KV line is under construction from Barapukuria in the upper part of the Northern region of Bangladesh to Sirajganj near the Jamuna River. Similarly a 230 KV line is under construction from Ishurdi in the northern region to Khulna in the Western region of Bangladesh. In the same way a 132 KV system interconnects the Northern and Western regions in the western part of the country.
The paragraphs above summarize the structure of the present transmission system. This system currently experiences problems of low voltage levels not only in the Dhaka area but also in the other regions of the country. PGCB is well aware of these problems and has taken steps to initiate a program of reactive compensation by way of adding shunt capacitors at different locations of the transmission system.
Table 2-6 summarizes the existing transmission lines. Table 2-7 summarizes the 230/132 KV transformers.
2.3.2 Committed Transmission Projects
Additionally, we include committed transmission facilities in the transmission planning process. These are facilities that are not actually operating as part of the existing transmission system, but that are far enough along in the process of approving, financing, and building that it is highly likely that it will be built and become operational. A list of committed transmission facilities is shown in Table 2-8.
Table 2-6 Existing Transmission Lines
Region | Voltage, Nominal kV | Length, Circuit-Km |
Southern | 230 | 623 |
| 132 | 1326 |
Dhaka | 230 | 673 |
| 132 | 597 |
Central | 132 | 804 |
Western | 230 | 140 |
| 132 | 990 |
Northern | 132 | 1151 |
| | |
Total | 230 | 1436 |
| 132 | 4868 |
Table 2-7 Existing 230/132 KV Transformers
Region | Capacity (MVA) |
Southern | 675 |
Dhaka | 2800 |
Northern | 450 |
| |
Total | 3925 |
Table 2-8 Committed Transmission Projects
SL No. | Name of Line, Substation and Capacitor Bank to be Added | Bus ID | Length / Capacity | Expected Year of Addition | Status |
1 | Saidpur-Barapukuria 132 kV double circuit line | 1425_1442 | 35 km | 2006 | Under Construction |
2 | Barapukuria-Bogra New 230 kV double circuit line | 2042_2040 | 105 km | 2007 | ” |
3 | 230/132 kV substation at Barapukuria | 2042_1442 | 2x225 MVA | 2006 | ” |
4 | 230/132 kV substation at Bogra New | 2040_1440 | 2x225 MVA | 2007 | ” |
5 | Bogra New-Bogra 132 kV double circuit line | 1440_1415 | NA | 2007 | ” |
6 | Bogra New -Sirajganj 230 kV double circuit line and Sirajganj 230 kV switching station | 2040_2036 | NA | 2007 | ” |
7 | Sirajganj-Baghabari 230 kV double circuit line | 2036_2030 | 55 km | 2007 | ” |
8 | Baghabari 230/132 kV substation | 2030_1412 | 2x225 MVA | 2007 | ” |
9 | Sirajganj-Ashuganj 230 kV double circuit line | 2036_2008 | 142 km | 2007 | ” |
10 | Baghabari-Ishurdi 230 kV double circuit line | 2030_2020 | 55 km | 2007 | ” |
11 | Ishurdi-Bheramara 230 kV double circuit line | 2020_2044 | 10 km | 2007 | ” |
SL No. | Name of Line, Substation and Capacitor Bank to be Added | Bus ID | Length / Capacity | Expected Year of Addition | Status |
12 | Bheramara-Khulna South 230 kV double circuit line | 2044_2032 | 157 km | 2007 | ” |
13 | Khulna South 230/132 kV substation | 2032_1332 | 2x225 MVA | 2007 | ” |
14 | Naogaon-Niamotpur 132 kV single circuit line | 1417_1404 | 48 km | 2007 | ” |
15 | 132/33 kV substation at Niamotpur | 1404 | 2x15/20 MVA | 2007 | ” |
16 | Natore-Rajshahi 132 kV single circuit line | 1403_1405 | 40 km | 2006 | ” |
17 | Khulna Central-Khulna South 132 kV double circuit line | 1302_1332 | NA | 2007 | ” |
18 | Khulna South-Satkhira 132 kV double circuit line | 1332_1334 | 60 km | 2006 | ” |
19 | 132/33 kV substation at Satkhira | 1334 | 2x25/41 MVA | 2006 | ” |
20 | Khulna South-Gallamari 132 kV double circuit line | 1332_1329 | NA | 2009 | Committed |
21 | 132/33 kV substation at Gallamari | 1329 | 2x25/41 MVA | 2009 | ” |
22 | Madaripur-Gopalganj 132 kV double circuit line | 1315_1314 | 37 km | 2006 | Under Construction |
23 | 132/33 kV substation at Gopalganj | 1314 | 2x25/41 MVA | 2006 | ” |
24 | Joydebpur-Kabirpur 132 kV double circuit line | 1132_1126 | 15 km | 2006 | ” |
25 | Kabirpur-Tangail 132 kV single circuit line | 1126_1128 | 50 km | 2006 | ” |
26 | Uttara 132/33 kV substation | 1123 | NA | 2006 | ” |
27 | Bashundhara-Jamuna Group 132 kV single circuit line | 1124_1144 | NA | 2006 | ” |
28 | Jamuna Group 132/33 kV substation | 1144 | NA | 2006 | ” |
29 | Rampura-Gulshan 132 kV double circuit U/G cable | 1107_1116 | 3 km | 2007 | ” |
30 | 132/33 kV substation at Gulshan | 1116 | 2x80/120 MVA | 2007 | ” |
31 | Kamrangirchar 132/33 kV substation | 1118 | 2x50/75 MVA | 2007 | ” |
32 | Sitalakhya-Munshiganj 132 kV double circuit line | 1114_1117 | 6 km | 2009 | Committed |
33 | 132/33 kV substation at Munshiganj | 1117 | 2x50/75 MVA | 2009 | ” |
34 | Matuail 132/33 kV substation | 1109 | 2x50/75 MVA | 2006 | Under Construction |
35 | Meghnaghat 132/33 kV substation | 1115 | 2x50/75 MVA | 2009 | Committed |
36 | Daudkandi 132/33 kV substation | 1033 | 2x50/75 MVA | 2009 | ” |
37 | B. Baria 132/33 kV substation | 1210 | 2x25/41MVA | 2009 | ” |
38 | Kulshi-Halishahar 132 kV double circuit line | 1013_1011 | 13 km | 2007 | Under Construction |
39 | Kulshi-Bakulia-Sikalbaha 132 kV double circuit line | 1013_1016_1006 | NA | 2006 | ” |
40 | 132/33 kV substation at Bakulia | 1016 | 2x48/64MVA | 2006 | ” |
41 | Sikalbaha-Shahmirpur-Juldah 132 kV double circuit line | 1006_1018_1017 | NA | 2006 | ” |
42 | 132/33 kV substation at Shahmirpur | 1018 | 2x48/64MVA | 2006 | ” |
SL No. | Name of Line, Substation and Capacitor Bank to be Added | Bus ID | Length / Capacity | Expected Year of Addition | Status |
43 | 132/33 kV substation at Juldah | 1017 | 2x48/64MVA | 2006 | ” |
44 | Addition of third 230/132 kV transformer at Aminbazar | 2034_1134 | 1x225 MVA | 2007 | Committed |
45 | Addition of third 230/132 kV transformer at Rampura | 2016_1107 | 1x225 MVA | 2007 | ” |
46 | Addition of third 230/132 kV transformer at Haripur | 2012_1101 | 1x225 MVA | 2007 | ” |
47 | Addition of second 230/132 kV transformer at Comilla (N) | 2005_1030 | 1x225 MVA | 2009 | ” |
48 | Addition of fourth 230/132 kV transformer at Hathazari | 2002_1003 | 1x225 MVA | 2009 | ” |
49 | Meghnaghat-Aminbazar 400 kV double circuit line | 4014-4034 | 48 km | 2010* | ” |
50 | Old Airport 230 kV Substation | 2045 | 3x225 MVA | 2010 | ’’ |
51 | Aminbazar-Old Airport 230 kV double circuit line | 2034-2045 | NA | 2010 | ’’ |
52 | Ullon-Rampura 132 kV double circuit underground cable | 1105-1107 | 4 km | 2010 | ’’ |
53 | Naogaon-Joypurhat 132 kV line | 1417-1416 | 40 kM | 2010 | ’’ |
54 | Thakugaon-Panchaghar 132 kV line | 1432-1431 | 45 km | 2010 | ’’ |
55 | Chuadanga-Jhenida-Magura 132 kV line | 1304-1307-1309 | 73 km | 2010 | ’’ |
56 | Four 132/33 kV Substations one each at Chuadanga, Magura, Joypurhat and Panchaghar | 1304, 1309, 1416, 1431 | NA | 2010 | ’’ |
57 | Capacitor Bank at Rampura | 1107 | 2x45 MVAR | 2007 | ” |
58 | Capacitor Bank at Aminbazar | 1134 | 2x45 MVAR | 2007 | ” |
59 | Capacitor Bank at Hathazari | 1003 | 1x45 MVAR | 2007 | ” |
60 | Capacitor Bank at Dohazari | 1008 | 1x45 MVAR | 2007 | ” |
61 | Capacitor Bank at Bakulia | 1016 | 1x45 MVAR | 2007 | ” |
62 | Capacitor Bank at Barisal | 1320 | 1x45 MVAR | 2007 | ” |
63 | Capacitor Bank at Jessore | 1306 | 1x45 MVAR | 2007 | ” |
64 | Capacitor Bank at Madaripur | 1315 | 1x45 MVAR | 2007 | ” |
* Initially operated at 230 kV. NA Not Available
Section 3 Load Forecast
3.1 INTRODUCTION
The purpose of this report is to present the work performed in developing the load forecast for Bangladesh. The load forecast is the key input for the generation and transmission analysis, and this report presents our findings.
Nexant developed forecasts of maximum demand (MW) and sales (GWH) for the 2005–2025 period. This forecasting period matches the Master Plan time period. Necessary input data for this task was obtained and compiled using Government of Bangladesh (GOB) and utility sources including BPDB, PGCB, Dhaka Electric Supply Authority (DESA), Dhaka Electric Supply Company (DESCO) and Rural Electrification Board (REB). Interviews were also conducted with representatives from major consumers groups such as the Federation of Bangladesh Chambers of Commerce and Industry. Data include historical sales and demand data, economic data for Bangladesh, and economic development targets and projections. We also conducted a high-level market study to collect data and expert opinions about the adequacy and reliability of electricity supply, and to get information about the future load growth.
3.2 LOAD AND GDP ANALYSIS
3.2.1 Historical Sales and Demand Data
The first step in our analysis was to review historical electricity consumption data.
Electricity consumption has been consistently growing at a relatively high rate (more then 8% annually) and generation resources were continuously inadequate to supply this increased load. As the result, every year in the last decade has some level of recorded capacity and corresponding energy shortage that is not reflected in the historical energy demand numbers.
Table 3-1 presents the electricity energy balance overview for each utility and for the entire interconnected power system. Total sales figures for each utility are the sum of the sales of each customer class. Energy imported by each utility was derived from utility statistics.
Based on sales and imported energy we calculated distribution losses. (Note: for DESA this includes losses in DESA’s transmission system.) We also calculated average distribution losses based on the overall energy imports and sales. Average distribution losses show significant improvement (loss reduction) coming down from 30% a decade ago to around 20% in the last year. Total imported energy for all utilities was then compared with the system net energy generation. The column labeled “Net Generation (GWH)” refers to inputs to the transmission system at the high side of power plant main transformers. This is less than generator output by the amount of energy consumption in plant auxiliaries and main transformer. From these two numbers we were able to calculate transmission losses.
Transmission losses also show a steady reduction over time.
Table 3-1 Historical Sales and Loss Data by Utility
Year | Company | Energy Sales by Customer Class (GWh) | Distrib. Loss (%) | Imported Energy (GWh) | Transm. Loss (%) | Net Generation (GWh) |
Residential | Agricaltural | Commercial | Industrial | Other | Total Sales |
| BPDB | 1181.3 | 98.0 | 315.5 | 1303.7 | 123.4 | 3021.8 | 30.7% | 4361.5 | 4.7% | 9221.1 |
| DESA | 889.2 | 13.4 | 199.7 | 1189.9 | 69.7 | 2361.9 | 32.9% | 3519.6 | | |
1994 | REB | 245.2 | 157.3 | 43.1 | 317.6 | 1.9 | 765.2 | 15.6% | 906.1 | | |
| TOTAL | 2315.7 | 268.7 | 558.3 | 2811.2 | 195.0 | 6148.8 | 30.0% | 8787.2 | | |
| BPDB | 1232.1 | 145.0 | 305.9 | 1402.7 | 134.4 | 3220.2 | 29.9% | 4596.3 | 4.1% | 10166.3 |
| DESA | 1079.1 | 15.8 | 202.5 | 1294.3 | 72.7 | 2664.4 | 31.9% | 3913.5 | | |
1995 | REB | 322.9 | 273.3 | 57.5 | 394.3 | 2.1 | 1050.1 | 15.1% | 1237.3 | | |
| TOTAL | 2634.1 | 434.1 | 565.9 | 3091.3 | 209.3 | 6934.7 | 28.9% | 9747.1 | | |
| BPDB | 1313.6 | 125.7 | 314.9 | 1468.7 | 139.7 | 3362.6 | 29.1% | 4742.1 | 4.2% | 10832.9 |
| DESA | 1238.6 | 15.5 | 200.7 | 1383.5 | 80.9 | 2919.3 | 31.5% | 4261.1 | | |
1996 | REB | 415.6 | 242.0 | 68.9 | 441.4 | 4.3 | 1172.2 | 14.6% | 1372.2 | | |
| TOTAL | 2967.7 | 383.2 | 584.6 | 3293.6 | 224.9 | 7454.0 | 28.2% | 10375.5 | | |
| BPDB | 1291.2 | 107.5 | 306.9 | 1519.9 | 135.4 | 3360.9 | 28.3% | 4686.2 | 4.2% | 11242.9 |
| DESA | 1455.5 | 10.1 | 206.4 | 1484.6 | 83.8 | 3240.5 | 29.8% | 4613.5 | | |
1997 | REB | 462.0 | 208.1 | 72.7 | 472.9 | 4.6 | 1220.3 | 17.1% | 1472.5 | | |
| TOTAL | 3208.7 | 325.8 | 585.9 | 3477.5 | 223.8 | 7821.7 | 27.4% | 10772.1 | | |
| BPDB | 1322.3 | 104.9 | 320.7 | 1602.8 | 133.7 | 3484.6 | 29.8% | 4965.3 | 4.4% | 12194.2 |
| DESA | 1641.3 | 8.4 | 202.7 | 1523.5 | 87.0 | 3462.9 | 30.4% | 4973.7 | | |
1998 | REB | 586.5 | 191.5 | 87.9 | 564.3 | 4.8 | 1434.9 | 16.5% | 1718.0 | | |
| TOTAL | 3550.1 | 304.8 | 611.3 | 3690.6 | 225.6 | 8382.4 | 28.1% | 11657.0 | | |
| BPDB | 1446.5 | 111.3 | 354.4 | 1667.3 | 146.4 | 3725.9 | 30.6% | 5365.5 | 4.7% | 13637.7 |
| DESA | 1722.9 | 4.4 | 195.8 | 1583.8 | 82.7 | 3589.6 | 30.8% | 5183.7 | | |
1999 | REB | 793.2 | 312.1 | 118.5 | 759.9 | 5.4 | 1989.2 | 18.6% | 2442.7 | | |
| TOTAL | 3962.5 | 427.9 | 668.7 | 4011.0 | 234.5 | 9304.7 | 28.4% | 12991.9 | | |
| BPDB | 1565.6 | 88.4 | 390.7 | 1835.8 | 160.5 | 4041.1 | 27.7% | 5591.6 | 4.9% | 14739.1 |
| DESA | 1471.4 | 1.1 | 171.1 | 1886.9 | 51.7 | 3582.1 | 31.7% | 5247.7 | | |
2000 | REB | 1005.2 | 262.2 | 149.5 | 1034.6 | 8.2 | 2459.5 | 22.5% | 3172.4 | | |
| TOTAL | 4042.2 | 351.7 | 711.3 | 4757.3 | 220.3 | 10082.8 | 28.0% | 14011.6 | | |
| BPDB | 1725.0 | 111.0 | 440.3 | 1968.8 | 174.8 | 4419.9 | 26.1% | 5981.9 | 4.2% | 16254.2 |
| DESA | 1639.3 | 0.9 | 167.0 | 2002.3 | 48.9 | 3858.4 | 32.5% | 5718.7 | | |
2001 | REB | 1230.5 | 370.9 | 180.6 | 1340.3 | 8.3 | 3130.6 | 19.0% | 3864.2 | | |
| TOTAL | 4594.8 | 482.8 | 787.9 | 5311.4 | 232.0 | 11408.9 | 26.7% | 15564.8 | | |
| BPDB | 1891.7 | 96.2 | 473.7 | 2090.5 | 184.2 | 4736.3 | 24.5% | 6273.4 | 3.8% | 17444.8 |
| DESA | 1691.5 | 0.7 | 159.5 | 1419.4 | 51.1 | 3410.2 | 36.6% | 5380.5 | | |
2002 | DESCO REB | 267.9 1659.9 | 0.0 357.2 | 23.8 219.4 | 185.8 1648.7 | 16.1 9.8 | 493.6 3894.9 | 25.2% 17.2% | 660.3 4466.2 | | |
| TOTAL | 5511.0 | 454.1 | 876.4 | 5344.3 | 261.2 | 12535.0 | 25.3% | 16780.4 | | |
| BPDB | 1993.7 | 75.3 | 497.4 | 2078.4 | 192.9 | 4837.7 | 22.4% | 6230.5 | 3.8% | 18422.1 |
| DESA | 1657.6 | 0.3 | 211.9 | 1547.0 | 52.7 | 3475.2 | 33.0% | 5184.6 | | |
2003 | DESCO REB | 348.0 2037.0 | 0.0 399.0 | 41.0 268.0 | 256.0 2173.0 | 31.0 11.0 | 676.0 4888.0 | 21.5% 14.1% | 861.4 5447.5 | | |
| TOTAL | 6036.3 | 474.6 | 1018.3 | 6054.4 | 287.7 | 13876.9 | 21.7% | 17724.0 | | |
| BPDB | 2066.7 | 78.8 | 504.7 | 2086.8 | 204.3 | 4941.2 | 21.3% | 6281.0 | 3.5% | 20062.1 |
| DESA | 1379.0 | 0.2 | 222.0 | 1529.0 | 48.0 | 3178.2 | 34.5% | 4854.0 | | |
2004 | DESCO REB | 678.0 2475.0 | 0.0 527.0 | 104.0 320.0 | 597.0 2469.0 | 29.0 14.0 | 1408.0 5805.0 | 19.1% 13.7% | 1740.8 6486.0 | | |
| TOTAL | 6598.7 | 606.0 | 1150.7 | 6681.8 | 295.3 | 15332.4 | 20.8% | 19361.8 | | |
3.2.2 Historical Net Peak Load
Table 3-2 presents historical net peak load values for the 1994-2004 period. Peak load is calculated by adding the net MW generation of all units operating at the time of peak demand. Generation by BPDB units is recorded as gross generation, before subtracting each unit’s auxiliary power consumption, whereas generation by independent power producer (IPP) units is recorded as net. The analysis was done by subtracting IPP net MW generation at time of peak from the total maximum generation, then recalculating BPDB values to reflect net MW generation. We used an average auxiliary load of 5.5% in this step. Finally both net MW generation components were added to arrive at the system net peak load.
Table 3-2 Net Peak Load Values
Fiscal Year | Max. Generation (PDB gross & IPP net) MW | PDB (net) MW | IPP (net) MW | Combined Peak Load MW |
1994 | 1875 | 1772 | | 1772 |
1995 | 1970 | 1862 | | 1862 |
1996 | 2087 | 1972 | | 1972 |
1997 | 2114 | 1998 | | 1998 |
1998 | 2136 | 2019 | | 2019 |
1999 | 2449 | 2052 | 278 | 2330 |
2000 | 2665 | 2184 | 354 | 2538 |
2001 | 3033 | 2208 | 696 | 2904 |
2002 | 3248 | 2371 | 739 | 3110 |
2003 | 3458 | 2148 | 1185 | 3333 |
2004 | 3622 | 2244 | 1247 | 3491 |
3.2.3 Load Shedding Data and Analysis
The next step in our analysis was to review historical load shedding data and, if necessary, propose revisions to load shedding inputs used to calculate system Peak Load and Generation. This analysis is presented in Tables 3-3 and 3-4.
Official recorded maximum MW load shedding values are presented in Table 3-4. However, by looking at the historical records it was clear that the maximum load shedding did not occur at the time of the total system peak load. So the maximum load shedding value should not simply be added to the recorded peak load.
In order to better estimate peak load, we analyzed daily load curves in winter months when there was no load shedding. From these curves we estimated the full value of the peak portion of the load curve. Next we analyzed load curves in peak load months. These curves were distorted, indicating load shedding. However, from these curves we were able to estimate the base component of the load. The peak and base components added together provided a more accurate estimate of peak load if there were no load shedding. Table 3-3 shows estimated peak load values and how they compare with the actual recorded net peak load values. The difference between estimated and actual peak load provides our revised estimate of the peak load shedding, also shown in Table 3-4.
Table 3-3 Peak Load Analysis
Fiscal Year | Estimated Gross Base Load (MW) | Est. Gross Peak Over Base Load (MW) | Estimated Gross Peak Load (MW) | Actual Net/Gross | Estimated Net Peak Load (MW) | Growth (%) | Actual Net Peak Load (MW) | Growth (%) |
1994 | 1,350 | 650 | 2,000 | 0.95 | 1,890 | | 1,772 | |
1995 | 1,450 | 700 | 2,150 | 0.95 | 2,032 | 7.5% | 1,862 | 5.1% |
1996 | 1,550 | 750 | 2,300 | 0.95 | 2,174 | 7.0% | 1,972 | 5.9% |
1997 | 1,725 | 800 | 2,525 | 0.95 | 2,386 | 9.8% | 1,998 | 1.3% |
1998 | 1,900 | 850 | 2,750 | 0.95 | 2,599 | 8.9% | 2,019 | 1.1% |
1999 | 2,100 | 900 | 3,000 | 0.95 | 2,854 | 9.1% | 2,330 | 15.4% |
2000 | 2,200 | 950 | 3,150 | 0.95 | 3,000 | 5.0% | 2,538 | 8.9% |
2001 | 2,300 | 1,025 | 3,325 | 0.96 | 3,184 | 5.6% | 2,904 | 14.4% |
2002 | 2,450 | 1,100 | 3,550 | 0.96 | 3,399 | 6.8% | 3,110 | 7.1% |
2003 | 2,600 | 1,200 | 3,800 | 0.96 | 3,663 | 7.0% | 3,333 | 7.2% |
2004 | 2,850 | 1,250 | 4,100 | 0.96 | 3,952 | 7.9% | 3,491 | 4.7% |
Table 3-4 Load Shedding Analysis
Fiscal Year | Net Energy Generation (GWh) | Recorded Load Shedding (GWh) | Est. Load Shedding(GWh) | Est. Net Energy Generation (GWh) | Net Peak Load (MW) | Rec. Max Load Shedding(MW) | Est. Load Shedding(MW) | Estimated Net Peak Load (MW) | EstimatedLoad Factor (%) |
1994 | 9,221 | 99 | 148 | 9,369 | 1,772 | 540 | 118 | 1,890 | 56.6% |
1995 | 10,166 | 87 | 130 | 10,296 | 1,862 | 537 | 170 | 2,032 | 57.9% |
1996 | 10,833 | 500 | 750 | 11,583 | 1,972 | 545 | 202 | 2,174 | 60.8% |
1997 | 11,243 | 550 | 826 | 12,069 | 1,998 | 674 | 388 | 2,386 | 57.7% |
1998 | 12,194 | 516 | 774 | 12,968 | 2,019 | 711 | 580 | 2,599 | 57.0% |
1999 | 13,638 | 264 | 396 | 14,034 | 2,330 | 774 | 524 | 2,854 | 56.1% |
2000 | 14,739 | 121 | 182 | 14,921 | 2,538 | 536 | 462 | 3,000 | 56.8% |
2001 | 16,254 | 119 | 178 | 16,432 | 2,904 | 663 | 280 | 3,184 | 58.9% |
2002 | 17,445 | 70 | 105 | 17,549 | 3,110 | 367 | 289 | 3,399 | 58.9% |
2003 | 18,422 | 69 | 104 | 18,526 | 3,333 | 468 | 330 | 3,663 | 57.7% |
2004 | 20,062 | 147 | 221 | 20,283 | 3,491 | 694 | 461 | 3,952 | 58.6% |
Recorded energy load shedding was also revised to more accurate values. Energy shedding has much less influence on the results, so we made a simpler estimate of net energy generation before load shedding. We added another half of the recorded GWH value to account for the portion of the load not served as the result of low voltage and distribution system constraints.
The estimated load factors shown in Table 3-4 were calculated based on the newly estimated net peak load and net energy generation values.
3.2.4 Distribution of Load by Region
One of the tasks in developing the load forecast was to estimate the future distribution of load by region. We use the regional distribution of load to estimate the future demand at each transmission substation, which the transmission planning process requires. Peak demand is more important than energy demand for transmission planning purposes, but data on peak demand by region was not available. Therefore we used energy demand to estimate regional demand, a reasonable approach when load shape is similar throughout the country.
For power system development analysis, Bangladesh is divided into five geographical regions: the Central, Northern, Southern, Western and greater Dhaka regions. Our approach was to collect historical sales data by region, and conduct analysis of historical load
distribution factors. The load distribution factor for a region is that region’s percentage of total national demand. This analysis is summarized in Table 3-5.
The anomaly in the last year, 2004, occurred because a large portion of DESA load in the Dhaka region was transferred to REB. In effect a large area surrounding Dhaka, but not within the municipality, was transferred from one region to another, and then treated administratively as being in the Central region. This significantly altered load distribution factors for those two regions. However, the sum of the load distribution factors of those two regions was nearly the same in 2004 as in all the other years. The apparent shift in load distribution was only administrative. The loads in the Dhaka and Central regions continued to be supplied by the same transmission substations, as they will in the future. Thus for transmission planning purposes there was no significant shift in load distribution factors in 2004.
With this explanation in mind, Table 3-5 clearly shows minimal variations in load distribution factors over the period 1997 – 2004. This shows that all regions grew at about the same rate. We assume that this will continue and all regions will continue to grow at the same rate. For transmission planning purposes, individual substations within a region may grow at different rates based on knowledge of local conditions. However, the sum of demand at all transmission substations in a region is constrained to grow at the regional rate. The implication is that demand may shift between substations within a region, but may not shift between substations in two different regions. For later analysis we use the 2003 regional load distribution factors and designation of which substations are part of each region. However, because we forecast all regions to grow at the same rate, the linking of substations to regions makes little difference.
Table 3-5 Historical Electricity Requirements by Region and Distribution Factors
| Imported Energy (GWh) |
Region | 1997 | 1998 | 1999 | 2000 | 2001 | 2002 | 2003 | 2004 |
Central | 909 | 981 | 1121 | 1172 | 1290 | 1408 | 1519 | 3642 |
Northern | 1392 | 1471 | 1635 | 1728 | 1957 | 2044 | 2136 | 2417 |
Southern | 2170 | 2362 | 2688 | 2802 | 3168 | 3449 | 3617 | 3919 |
Western | 1327 | 1414 | 1632 | 1746 | 1898 | 2023 | 2121 | 2306 |
Dhaka | 4962 | 5419 | 5949 | 6504 | 7241 | 7846 | 8320 | 7071 |
Total | 10760 | 11647 | 13024 | 13952 | 15554 | 16770 | 17714 | 19353 |
Region | Distribution by Region (%) |
Central | 8.4% | 8.4% | 8.6% | 8.4% | 8.3% | 8.4% | 8.6% | 18.8% |
Northern | 12.9% | 12.6% | 12.6% | 12.4% | 12.6% | 12.2% | 12.1% | 12.5% |
Southern | 20.2% | 20.3% | 20.6% | 20.1% | 20.4% | 20.6% | 20.4% | 20.2% |
Western | 12.3% | 12.1% | 12.5% | 12.5% | 12.2% | 12.1% | 12.0% | 11.9% |
Dhaka | 46.1% | 46.5% | 45.7% | 46.6% | 46.6% | 46.8% | 47.0% | 36.5% |
Total | 100.0% | 100.0% | 100.0% | 100.0% | 100.0% | 100.0% | 100.0% | 100.0% |
3.2.5 Historical GDP Data
Gross domestic product (GDP) was historically used as the best proxy to link electricity demand with economic activity in many developing counties. For many countries and over long periods of time, overall electricity demand is closely linked to growth in GDP.
During the last 10 years Bangladesh’s economy has regained pace and GDP grew at a consistent rate. Increased economic activity, reflected in the GDP growth, is the key driver
behind the increase in the electricity demand. Historical GDP values are presented in Table 3-
6. Compound average annual GDP growth over the last 11 years (1994-2004 period) is 5.1%. This compares with the average annual net energy generation growth rate of 8.1% over the same period
Table 3-6 Historical GDP and Growth Rates, Constant 1995-6 Taka
Fiscal Year | GDP (million Taka) | GDP Growth (%) |
1994 | 1,515,139 | |
1995 | 1,589,762 | 4.93% |
1996 | 1,663,240 | 4.62% |
1997 | 1,752,847 | 5.39% |
1998 | 1,844,478 | 5.23% |
1999 | 1,934,291 | 4.87% |
2000 | 2,049,276 | 5.94% |
2001 | 2,157,353 | 5.27% |
2002 | 2,252,609 | 4.42% |
2003 | 2,371,006 | 5.26% |
2004 | 2,501,813 | 5.52% |
3.2.6 GDP Growth Forecast
In order to be able to use GDP as an input for future load forecasting, we had to develop forecasts for growth rates for the entire planning period, for the three different growth scenarios. In all the scenarios we assume continued robust growth in Bangladesh’s economy. As the economy becomes larger, we assume that economic growth is more difficult to sustain. Therefore the growth rates are higher in the early years than in the later years. The Base Case assumes initial growth over the period 2005 – 2015 slightly higher than the 1994 – 2004 compound annual growth rate. From 2016 – 2025 the growth rates are slightly below the 1994 – 2004 rates. Low Case growth rates average about 0.7 percentage point lower than the Base Case. For the High Case we use current GOB forecast GDP growth rates that average about 2.7 percentage points higher than the Base Case.
Table 3-7 presents our GDP forecasts. The Base Case starts with the Asian Development Bank (ADB) forecast growth rates for Bangladesh of 5.3% for 2005 and 6.0% for 2006. We assumed growth of 6% for four more years, then gradually declining by 0.5% of the each following five year period as the economy expands, ending with a 4.5% growth rate for the last five year period 2021 – 2025.
For the High Case the GOB assumed a more optimistic initial growth rate of 6.5% for 2005. The rate increases steadily to 9% in 2015 and 2016, then falls steadily to 7% by 2025.
For the Low Case, we started with a growth rate of 5.3% for 2005, then 5% for the next five years, slightly lower than the historical growth rate for 1994 – 2004. Growth drops to 4.5% for the next ten years, then to 4.5% for the final five year period.
Table 3-7 GDP Projections and Growth Rates, Constant 1995-6 Taka
| Base Case | High Case | Low Case |
Fiscal Year | GDP (million Taka) | Growth Rate | GDP (million Taka) | Growth Rate | GDP (million Taka) | Growth Rate |
2005 | 2,634,409 | 5.3% | 2,664,431 | 6.5% | 2,634,409 | 5.3% |
2006 | 2,792,474 | 6.0% | 2,850,941 | 7.0% | 2,766,130 | 5.0% |
2007 | 2,960,022 | 6.0% | 3,050,507 | 7.0% | 2,904,436 | 5.0% |
2008 | 3,137,623 | 6.0% | 3,264,042 | 7.0% | 3,049,658 | 5.0% |
2009 | 3,325,881 | 6.0% | 3,508,846 | 7.5% | 3,202,141 | 5.0% |
2010 | 3,525,434 | 6.0% | 3,789,553 | 8.0% | 3,362,248 | 5.0% |
2011 | 3,719,332 | 5.5% | 4,092,717 | 8.0% | 3,513,549 | 4.5% |
2012 | 3,923,896 | 5.5% | 4,440,598 | 8.5% | 3,671,659 | 4.5% |
2013 | 4,139,710 | 5.5% | 4,818,049 | 8.5% | 3,836,883 | 4.5% |
2014 | 4,367,394 | 5.5% | 5,227,583 | 8.5% | 4,009,543 | 4.5% |
2015 | 4,607,601 | 5.5% | 5,698,066 | 9.0% | 4,189,972 | 4.5% |
2016 | 4,837,981 | 5.0% | 6,210,892 | 9.0% | 4,378,521 | 4.5% |
2017 | 5,079,880 | 5.0% | 6,738,818 | 8.5% | 4,575,555 | 4.5% |
2018 | 5,333,874 | 5.0% | 7,311,617 | 8.5% | 4,781,455 | 4.5% |
2019 | 5,600,568 | 5.0% | 7,933,105 | 8.5% | 4,996,620 | 4.5% |
2020 | 5,880,596 | 5.0% | 8,567,753 | 8.0% | 5,221,468 | 4.5% |
2021 | 6,145,223 | 4.5% | 9,253,173 | 8.0% | 5,430,327 | 4.0% |
2022 | 6,421,758 | 4.5% | 9,993,427 | 8.0% | 5,647,540 | 4.0% |
2023 | 6,710,737 | 4.5% | 10,742,934 | 7.5% | 5,873,441 | 4.0% |
2024 | 7,012,720 | 4.5% | 11,548,654 | 7.5% | 6,108,379 | 4.0% |
2025 | 7,328,292 | 4.5% | 12,357,060 | 7.0% | 6,352,714 | 4.0% |
Average growth | | 5.2% | | 8.0% | | 4.5% |
3.2.7 Demand Side Management, Load Management, and Energy Efficiency
Improving the typical load pattern of the Bangladesh national grid depicted below has long been a challenge for power system planners. The annual load factor of the grid is stands at 58%. The daily load curve is characterized by two peaks, one about 70% of the other. The smaller peak occurs during mid-morning, and the larger one during early evening. The difference between the morning and evening peak is currently about 1,000 MW. Serving this type of demand pattern requires peak load power generation facilities operating at expensive low capacity factors. This load factor has prevailed despite the growth of power demand in the country over the last decades.
It is never practical to achieve a technically ideal flat load curve for the electric utility.
BPDB took efforts in the past, to improve performance of the system by adopting measures to shift the peak, and to reduce capital investment on peak power generation. Lately, Power Cell has formulated two types of load management scenarios keeping in view of reducing electricity consumption during peak hours. Recommendations were submitted to the GOB accordingly. Load management in controlling the daily peak is a practice widely adopted by the utilities in neighboring countries.
The two types of management proposed by Power Cell are Load Side Management and Demand Side Management.
3.2.7.1 Load Side Management (LSM)
LSM is a deliberate scheduled program of load shedding from the system, as it transpires without interfering with economic activities. It is credited to increase the efficiency, reliability and quality of supply in the system by saving on expensive peak power production cost. This also tends to reduce the electricity tariff, which ultimately boosts up economic activities. The management also outlines staggering the usage time of different sectors of consumers, including staggering of holidays in industrial parks, or zones.
Power Cell has submitted a country wide detailed LSM program aimed at reducing demand by about 500 MW during peak periods. The program is estimated to free facilities to serve additional 250,000 domestic consumers.
During the month of March 2005, GOB constituted a committee comprising members from Power Cell and stakeholders for formulating various technical measures to accomplish economic load management. The following measures were adopted by the Committee:
− Holiday staggering in shopping and commercial centers
− Disconnecting illegal power connections
− Preventing use of electric heater for cooking in areas not served by piped gas.
− Switching off Bill Board and Street lighting during evening peak
− Discourage high level of illumination in shops and petrol pumps
− Switching off air conditioners during evening peak
− Discourage domestic water pump operation during peak
− Promote advertisement in mass media public for awareness
− Controlling demand by reducing system loss
− Installation of power factor improvement capacitor in distribution system
− Promoting use of energy efficient lighting, plant and machinery
− Keep the power plants in operation by ensuring proper maintenance
− Maintenance and expansion of power distribution facilities
− Promote advertisement in mass media for public awareness
− Updating long range demand forecast
− Project planning
− Addition of new generating plants in the system
− Expansion of transmission and distribution system
The proposed load management scheme outlines division of cities in 7 to 14 electrical zones, depending on size. Power will be shut down in all shopping centers of a zone, except one or two in a given city, during evening peak. This will allow people to carry out their emergency shopping, only in the privileged electrical zone during evening peak.
Power demand saved by load management and holiday staggering is calculated as follows:
- Total commercial load of the country – 220 MW
− 70% of this demand under management equals to 154 MW
- Total industrial load of the country – 1271 MW
− 40% of this demand under management equals to 508 MW
- Total irrigation load of the country – 115 MW
− 90% of this demand under management equals to 103 MW
The total demand shifted from peak by such management is calculated 765 MW
3.2.7.2 Demand Side Management (DSM)
Energy Efficiency
One aspect of DSM is aimed at reducing the system demand by using energy efficient devices in place of conventional types. A study conducted by Power Cell emphasized the use of energy efficient lamps, substituting electromagnetic ballast with electronic ballast for fluorescent lamps, and choke type ceiling fan speed regulators with electronic type. These steps would result in substantial saving in both power and energy demand.
The domestic power demand is figured at 1,136 MW. It is estimated that switching to compact fluorescent type lamp (CFL) and fluorescent lamp for domestic lighting alone could save about 355 MW. No estimate has been made on the saving of power by switching from conventional electromagnetic choke type to the power-saving electronic type fan regulator, though savings would likely be significant.
Another study showed that 12 large offices in Dhaka city, such as the Prime Minister’s office, Member of Parliament Hostel, Secretariat, Planning Commission, government hospital and Bangabhaban, consume a total of 41 GWH per annum. This could be reduced to 7 GWH by using CFL type lamp. Pursuant to the above, a Cabinet Division decision was taken on the use of energy efficient CFL lamps in all government offices.
Energy Leakage and Load Management
Bangladesh has been dealing with very high system losses for many years. Reduction of the technical component of loss would require large investments involving upgrade and reinforcement of the transmission and distribution systems. On the other hand, reduction of the non-technical component of the loss could be achieved, with good management and small investment on supportive hardware, such as meters and test instruments. Poor management, weak administration, undisciplined employees, corruption at both utility and consumer levels, and lack of firm political support were identified as factors contribution to high non-technical loss in the power sector.
To improve the situation of energy leakage and power theft, a well designed metering system is needed. Establishing some standards and implementing and enforcing a system of penalties should reduce the non-technical losses (energy leakage) of the system. Power Cell has prepared a program on these issues that has been accepted and implemented by DESCO and DESA. The resultant benefit of the program implemented throughout the country is estimated at reducing about 500 MW demand in the system.
Consumer awareness and education are two important aspects of the program. Advertisement can play a big role. No assessment has however, been made to measure the motivation and extent of consumer acceptability, on the high investment cost of switching to energy efficient electronic solutions for their household need. The general public tends to ignore savings on device operation, who are more concerned with initial investment. Success of the management scheme largely depends on conveying correct messages to all consumers.
In our forecast of demand we do not take LSM or DSM specifically into account. Some amount of DSM (mostly informal) existed in the past, over the period when both load and GDP were growing. Thus some degree of DSM is implicitly incorporated in the correlation of GDP and load to be developed in the next subsection. Using that correlation implicitly incorporates a similar level of ongoing DSM activity.
We do not specifically account for price elasticity’s impact on demand. Some factors may reduce prices: more efficient generating units and transmission system, GOB’s goal of least cost planning and increasing the sector's efficiency, etc. But other goals of progressively implementing cost-reflective tariffs are probably more significant. Again we take price elasticity into account only to the extent that historical demand has been achieved during times when prices were increasing.
3.3 FORECASTING METHODOLOGY
To confirm that using GDP values is appropriate for load forecasting in Bangladesh, we first had to analyze the historical pattern of load growth vs. the GDP growth.
3.3.1 Regression Analysis
The goal of this part of the analysis was to find the historical correlation between GDP and electricity demand. In case satisfactory correlation is found, then the next step would be to conduct regression analysis to determine future demand based on projected GDP growth data.
Tables 3-3 and 3-4 show that estimated net peak load and estimated net energy generation show exponential growth. Table 3-6 indicates that GDP also increases exponentially over time. Therefore we first calculated and plotted the natural logarithm of the values for GDP and estimated net energy generation in GWH. Figure 3-1 presents the results. Figure 3-1 indicates strong correlation, so we used a computer-based regression analysis package to conduct full regression analysis. The results confirmed very high correlation with the following key indicators:
R2 = 0.995 and Standard Error = 0.018
Figure 3-1 Historical GDP and Net Energy Generation Correlation Graph
3.3.2 Energy Forecasting
As the result of the regression analysis, we were able to derive the regression formula that links GDP values with estimated net energy generation in the following form:
Ln (Estimated net energy generation (n)) = - 11.919 + 1.482 Ln (GDP (n)) where n = fiscal year
Using these results we proceed with forecasting future generation using the estimated GDP values in Section 2-6.
3.3.3 Peak Demand Forecasting
Based on the estimated load factor presented in Table 3-4, we then estimated how load factor might change over time. Using load factor to link net energy generation with peak demand forecasts is commonly used in planning studies. Load factor is calculated using the following formula:
Load Factor = Energy (MWH)/(Peak Demand (MW)*Time (Hours))
where Time (Hours) for our annual analysis equals 8,760 hours. Historical values are graphically presented in Figure 3-2. Next using regression analysis we calculated a trend line that extended 20 years in the future. This analysis provided a forecast of load factor during the study period. Since load factor did not change much during the last 10 years, the trend line shows just a slight increase over time.
Figure 3-2 Load Factor Analysis
Projected peak demand is forecast using the energy forecast and future load factor projection rearranging the earlier formula into:
Peak Load (MW) = Energy (MWH)/(Load Factor*Time (Hours))
3.4 FORECASTING RESULTS
Using the methodology and formulas described in Section 3, we developed electricity load forecasts for net peak load and net energy generation. This section presents the results of our load forecasting analysis. For reference, the 2004 values were 3,952 MW for estimated net peak load and 20,062 GWH for estimated net energy generation.
Table 3-8 includes Net Energy Generation and Net Peak Load forecasts corresponding to the three GDP growth scenarios. The load factor projection is the result of the trending analysis, gradually increasing from 58.2% to 59.2% over the entire planning period. Table 3-9 presents yearly and average compound growth rates over the 2004 – 2025 period for all cases.
Figures 3-3 and 3-4 graphically present the Net Energy Generation and Net Peak Load forecasts, first for the Base Case and then for the High and Low Cases.
Table 3-8 Net Energy Generation and Net Peak Load Forecasts
| Base Case | High Case | Low Case | Projected Load Factor |
Fiscal Year | Net Generation (GWh) | Net Peak Load (MW) | Net Generation (GWh) | Net Peak Load (MW) | Net Generation (GWh) | Net Peak Load (MW) |
2005 | 21,964 | 4,308 | 22,336 | 4,381 | 21,964 | 4,308 | 58.2% |
2006 | 23,945 | 4,693 | 24,692 | 4,839 | 23,611 | 4,627 | 58.2% |
2007 | 26,106 | 5,112 | 27,297 | 5,345 | 25,382 | 4,970 | 58.3% |
2008 | 28,461 | 5,569 | 30,177 | 5,904 | 27,286 | 5,339 | 58.3% |
2009 | 31,028 | 6,066 | 33,592 | 6,567 | 29,333 | 5,734 | 58.4% |
2010 | 33,828 | 6,608 | 37,652 | 7,355 | 31,533 | 6,160 | 58.4% |
2011 | 36,622 | 7,148 | 42,202 | 8,237 | 33,659 | 6,569 | 58.5% |
2012 | 39,647 | 7,732 | 47,627 | 9,288 | 35,928 | 7,007 | 58.5% |
2013 | 42,922 | 8,364 | 53,749 | 10,473 | 38,351 | 7,473 | 58.6% |
2014 | 46,467 | 9,047 | 60,659 | 11,810 | 40,937 | 7,970 | 58.6% |
2015 | 50,306 | 9,786 | 68,924 | 13,408 | 43,697 | 8,501 | 58.7% |
2016 | 54,079 | 10,512 | 78,316 | 15,223 | 46,643 | 9,066 | 58.7% |
2017 | 58,135 | 11,291 | 88,384 | 17,166 | 49,788 | 9,670 | 58.8% |
2018 | 62,496 | 12,128 | 99,746 | 19,357 | 53,145 | 10,313 | 58.8% |
2019 | 67,183 | 13,027 | 112,568 | 21,827 | 56,728 | 11,000 | 58.9% |
2020 | 72,222 | 13,993 | 126,172 | 24,445 | 60,553 | 11,732 | 58.9% |
2021 | 77,092 | 14,924 | 141,419 | 27,377 | 64,178 | 12,424 | 59.0% |
2022 | 82,290 | 15,917 | 158,510 | 30,661 | 68,020 | 13,157 | 59.0% |
2023 | 87,839 | 16,977 | 176,448 | 34,103 | 72,092 | 13,934 | 59.1% |
2024 | 93,761 | 18,107 | 196,415 | 37,931 | 76,408 | 14,756 | 59.1% |
2025 | 100,083 | 19,312 | 217,137 | 41,899 | 80,982 | 15,626 | 59.2% |
Table 3-9 Yearly and Average Growth Rates for Energy and Peak Load
| Base Case | High Case | Low Case |
Fiscal Year | Energy Growth Rate (%) | Peak Load Growth Rate (%) | Energy Growth Rate (%) | Peak Load Growth Rate (%) | Energy Growth Rate (%) | Peak Load Growth Rate (%) |
2005 | 8.3% | 9.0% | 10.1% | 10.9% | 8.3% | 9.0% |
2006 | 9.0% | 8.9% | 10.5% | 10.5% | 7.5% | 7.4% |
2007 | 9.0% | 8.9% | 10.5% | 10.5% | 7.5% | 7.4% |
2008 | 9.0% | 8.9% | 10.5% | 10.5% | 7.5% | 7.4% |
2009 | 9.0% | 8.9% | 11.3% | 11.2% | 7.5% | 7.4% |
2010 | 9.0% | 8.9% | 12.1% | 12.0% | 7.5% | 7.4% |
2011 | 8.3% | 8.2% | 12.1% | 12.0% | 6.7% | 6.7% |
2012 | 8.3% | 8.2% | 12.9% | 12.8% | 6.7% | 6.7% |
2013 | 8.3% | 8.2% | 12.9% | 12.8% | 6.7% | 6.7% |
2014 | 8.3% | 8.2% | 12.9% | 12.8% | 6.7% | 6.7% |
2015 | 8.3% | 8.2% | 13.6% | 13.5% | 6.7% | 6.7% |
2016 | 7.5% | 7.4% | 13.6% | 13.5% | 6.7% | 6.7% |
2017 | 7.5% | 7.4% | 12.9% | 12.8% | 6.7% | 6.7% |
2018 | 7.5% | 7.4% | 12.9% | 12.8% | 6.7% | 6.7% |
2019 | 7.5% | 7.4% | 12.9% | 12.8% | 6.7% | 6.7% |
2020 | 7.5% | 7.4% | 12.1% | 12.0% | 6.7% | 6.7% |
2021 | 6.7% | 6.7% | 12.1% | 12.0% | 6.0% | 5.9% |
2022 | 6.7% | 6.7% | 12.1% | 12.0% | 6.0% | 5.9% |
2023 | 6.7% | 6.7% | 11.3% | 11.2% | 6.0% | 5.9% |
2024 | 6.7% | 6.7% | 11.3% | 11.2% | 6.0% | 5.9% |
2025 | 6.7% | 6.7% | 10.5% | 10.5% | 6.0% | 5.9% |
Average | 7.9% | 7.8% | 12.0% | 12.0% | 6.7% | 6.7% |
100,000 | | | | | | 25,000 |
90,000 | | | | | | |
80,000 | | | | | | 20,000 |
70,000 | | Energy | | | | |
60,000 | | | | | | 15,000 |
50,000 | | | | | | |
40,000 | | | | | | 10,000 |
30,000 | | | | Peak Load | | |
20,000 | | | | | | 5,000 |
10,000 | | | | | | |
- | | | | | | - |
2005 | 2007 2009 | 2011 2013 2015 | 2017 | 2019 2021 | 2023 2025 | |
Years |
Figure 3-3 Base Case Energy and Peak Load Forecasts
Figure 3-4 High and Low Case Energy and Peak Load Forecasts
Based on the load distribution factors from Table 3-5, we calculated the distribution of total load to each area. Tables 3-10 to 3-12 show the distributions for the Base, High, and Low Cases.
Table 3-13 presents estimated electricity sales for each area for the Base Case. In estimating sales numbers, a key assumption was how transmission and distribution losses will change over time. For transmission losses, we assumed that the 2004 loss value would continue for the next five years. Further improvements in the transmission network and the introduction of higher voltage lines, will result in transmission losses declining over time to reach the final 3% value in the year 2018 and thereafter.
All distribution utilities are continuously working to reduce distribution losses. We found that the goal for most of them is to completely eliminate non-technical losses and reduce distribution losses to approximately 10%. So we used 2004 average losses for each region as the starting point, with the gradual reduction to the final 10% loss value in 2019 and thereafter.
We chose to forecast net energy generation in part because we felt that in Bangladesh historical data on net energy generation was a more reliable measure of electricity demand than sales. We forecast net energy generation based on correlation with GDP. The above approach to forecasting sales in effect converts reduction in losses to sales, meaning that sales grow faster than net energy generation. Two factors justify this approach. First, there is little doubt that much of the distribution losses are non-technical. Reducing these losses means establishing metering and other procedures that more accurately determine the amount of service already actually delivered to consumers. Second, the correlation between GDP and net energy generation was derived during a period when losses were falling. Thus the impact on net energy generation of rising sales due to reduction of losses is incorporated in the basic correlation.
Table 3-10 Base Case Forecast Net Energy Generation and Net Peak Load by Region
| Central Region | Northern Region | Southern Region | Western Region | Dhaka Region |
Year | Net Generation (GWh) | Net Peak Load (MW) | Net Generation (GWh) | Net Peak Load (MW) | Net Generation (GWh) | Net Peak Load (MW) | Net Generation (GWh) | Net Peak Load (MW) | Net Generation (GWh) | Net Peak Load (MW) |
2005 | 1,884 | 369 | 2,649 | 519 | 4,485 | 880 | 2,630 | 516 | 10,317 | 2,024 |
2006 | 2,054 | 402 | 2,887 | 566 | 4,890 | 958 | 2,867 | 562 | 11,247 | 2,204 |
2007 | 2,239 | 438 | 3,148 | 616 | 5,331 | 1,044 | 3,126 | 612 | 12,262 | 2,401 |
2008 | 2,441 | 478 | 3,432 | 671 | 5,812 | 1,137 | 3,408 | 667 | 13,368 | 2,616 |
2009 | 2,661 | 520 | 3,742 | 731 | 6,336 | 1,239 | 3,715 | 726 | 14,574 | 2,849 |
2010 | 2,901 | 567 | 4,079 | 797 | 6,908 | 1,349 | 4,050 | 791 | 15,889 | 3,104 |
2011 | 3,141 | 613 | 4,416 | 862 | 7,478 | 1,460 | 4,385 | 856 | 17,202 | 3,357 |
2012 | 3,400 | 663 | 4,781 | 932 | 8,096 | 1,579 | 4,747 | 926 | 18,623 | 3,632 |
2013 | 3,681 | 717 | 5,176 | 1,009 | 8,765 | 1,708 | 5,139 | 1,001 | 20,161 | 3,929 |
2014 | 3,985 | 776 | 5,603 | 1,091 | 9,489 | 1,847 | 5,564 | 1,083 | 21,826 | 4,250 |
2015 | 4,315 | 839 | 6,066 | 1,180 | 10,273 | 1,998 | 6,023 | 1,172 | 23,629 | 4,597 |
2016 | 4,638 | 902 | 6,521 | 1,268 | 11,043 | 2,147 | 6,475 | 1,259 | 25,402 | 4,938 |
2017 | 4,986 | 968 | 7,010 | 1,362 | 11,871 | 2,306 | 6,961 | 1,352 | 27,307 | 5,304 |
2018 | 5,360 | 1,040 | 7,536 | 1,462 | 12,762 | 2,477 | 7,483 | 1,452 | 29,355 | 5,697 |
2019 | 5,762 | 1,117 | 8,101 | 1,571 | 13,719 | 2,660 | 8,044 | 1,560 | 31,557 | 6,119 |
2020 | 6,194 | 1,200 | 8,709 | 1,687 | 14,748 | 2,857 | 8,647 | 1,675 | 33,924 | 6,573 |
2021 | 6,612 | 1,280 | 9,296 | 1,800 | 15,742 | 3,048 | 9,230 | 1,787 | 36,211 | 7,010 |
2022 | 7,058 | 1,365 | 9,923 | 1,919 | 16,804 | 3,250 | 9,853 | 1,906 | 38,653 | 7,477 |
2023 | 7,534 | 1,456 | 10,592 | 2,047 | 17,937 | 3,467 | 10,517 | 2,033 | 41,259 | 7,974 |
2024 | 8,042 | 1,553 | 11,306 | 2,183 | 19,146 | 3,697 | 11,226 | 2,168 | 44,041 | 8,505 |
2025 | 8,584 | 1,656 | 12,069 | 2,329 | 20,437 | 3,944 | 11,983 | 2,312 | 47,010 | 9,071 |
Table 3-11 High Case Forecast Net Energy Generation and Net Peak Load by Region
| Central Region | Northern Region | Southern Region | Western Region | Dhaka Region |
Year | Net Generation (GWh) | Net Peak Load (MW) | Net Generation (GWh) | Net Peak Load (MW) | Net Generation (GWh) | Net Peak Load (MW) | Net Generation (GWh) | Net Peak Load (MW) | Net Generation (GWh) | Net Peak Load (MW) |
2005 | 1,916 | 376 | 2,693 | 528 | 4,561 | 895 | 2,674 | 525 | 10,491 | 2,058 |
2006 | 2,118 | 415 | 2,978 | 584 | 5,042 | 988 | 2,956 | 579 | 11,598 | 2,273 |
2007 | 2,341 | 458 | 3,292 | 645 | 5,574 | 1,092 | 3,268 | 640 | 12,822 | 2,511 |
2008 | 2,588 | 506 | 3,639 | 712 | 6,162 | 1,206 | 3,613 | 707 | 14,175 | 2,773 |
2009 | 2,881 | 563 | 4,051 | 792 | 6,860 | 1,341 | 4,022 | 786 | 15,779 | 3,085 |
2010 | 3,229 | 631 | 4,540 | 887 | 7,689 | 1,502 | 4,508 | 881 | 17,685 | 3,455 |
2011 | 3,619 | 706 | 5,089 | 993 | 8,618 | 1,682 | 5,053 | 986 | 19,823 | 3,869 |
2012 | 4,085 | 797 | 5,743 | 1,120 | 9,725 | 1,897 | 5,702 | 1,112 | 22,371 | 4,363 |
2013 | 4,610 | 898 | 6,481 | 1,263 | 10,976 | 2,139 | 6,435 | 1,254 | 25,247 | 4,920 |
2014 | 5,202 | 1,013 | 7,315 | 1,424 | 12,387 | 2,412 | 7,263 | 1,414 | 28,492 | 5,547 |
2015 | 5,911 | 1,150 | 8,311 | 1,617 | 14,074 | 2,738 | 8,252 | 1,605 | 32,375 | 6,298 |
2016 | 6,717 | 1,306 | 9,444 | 1,836 | 15,992 | 3,109 | 9,377 | 1,823 | 36,786 | 7,151 |
2017 | 7,580 | 1,472 | 10,658 | 2,070 | 18,048 | 3,505 | 10,582 | 2,055 | 41,515 | 8,063 |
2018 | 8,555 | 1,660 | 12,028 | 2,334 | 20,368 | 3,953 | 11,943 | 2,318 | 46,852 | 9,092 |
2019 | 9,655 | 1,872 | 13,574 | 2,632 | 22,987 | 4,457 | 13,478 | 2,613 | 52,875 | 10,253 |
2020 | 10,821 | 2,097 | 15,215 | 2,948 | 25,765 | 4,992 | 15,107 | 2,927 | 59,265 | 11,482 |
2021 | 12,129 | 2,348 | 17,053 | 3,301 | 28,878 | 5,590 | 16,932 | 3,278 | 66,427 | 12,859 |
2022 | 13,595 | 2,630 | 19,114 | 3,697 | 32,368 | 6,261 | 18,979 | 3,671 | 74,454 | 14,402 |
2023 | 15,133 | 2,925 | 21,277 | 4,112 | 36,031 | 6,964 | 21,126 | 4,083 | 82,880 | 16,019 |
2024 | 16,846 | 3,253 | 23,685 | 4,574 | 40,108 | 7,746 | 23,517 | 4,542 | 92,259 | 17,817 |
2025 | 18,623 | 3,593 | 26,184 | 5,052 | 44,340 | 8,556 | 25,998 | 5,017 | 101,992 | 19,680 |
Table 3-12 Low Case Forecast Net Energy Generation and Net Peak Load by Region
| Central Region | Northern Region | Southern Region | Western Region | Dhaka Region |
Year | Net Generation (GWh) | Net Peak Load (MW) | Net Generation (GWh) | Net Peak Load (MW) | Net Generation (GWh) | Net Peak Load (MW) | Net Generation (GWh) | Net Peak Load (MW) | Net Generation (GWh) | Net Peak Load (MW) |
2005 | 1,884 | 369 | 2,649 | 519 | 4,485 | 880 | 2,630 | 516 | 10,317 | 2,024 |
2006 | 2,025 | 397 | 2,847 | 558 | 4,821 | 945 | 2,827 | 554 | 11,090 | 2,174 |
2007 | 2,177 | 426 | 3,061 | 599 | 5,183 | 1,015 | 3,039 | 595 | 11,922 | 2,335 |
2008 | 2,340 | 458 | 3,290 | 644 | 5,572 | 1,090 | 3,267 | 639 | 12,817 | 2,508 |
2009 | 2,516 | 492 | 3,537 | 691 | 5,990 | 1,171 | 3,512 | 687 | 13,778 | 2,694 |
2010 | 2,704 | 528 | 3,802 | 743 | 6,439 | 1,258 | 3,775 | 737 | 14,811 | 2,893 |
2011 | 2,887 | 563 | 4,059 | 792 | 6,873 | 1,341 | 4,030 | 787 | 15,810 | 3,086 |
2012 | 3,081 | 601 | 4,332 | 845 | 7,337 | 1,431 | 4,302 | 839 | 16,876 | 3,291 |
2013 | 3,289 | 641 | 4,625 | 901 | 7,831 | 1,526 | 4,592 | 895 | 18,014 | 3,510 |
2014 | 3,511 | 684 | 4,936 | 961 | 8,359 | 1,628 | 4,901 | 954 | 19,228 | 3,744 |
2015 | 3,748 | 729 | 5,269 | 1,025 | 8,923 | 1,736 | 5,232 | 1,018 | 20,525 | 3,993 |
2016 | 4,000 | 778 | 5,625 | 1,093 | 9,525 | 1,851 | 5,585 | 1,086 | 21,909 | 4,259 |
2017 | 4,270 | 829 | 6,004 | 1,166 | 10,167 | 1,975 | 5,961 | 1,158 | 23,386 | 4,542 |
2018 | 4,558 | 885 | 6,409 | 1,244 | 10,852 | 2,106 | 6,363 | 1,235 | 24,963 | 4,844 |
2019 | 4,865 | 943 | 6,841 | 1,326 | 11,584 | 2,246 | 6,792 | 1,317 | 26,646 | 5,167 |
2020 | 5,193 | 1,006 | 7,302 | 1,415 | 12,365 | 2,396 | 7,250 | 1,405 | 28,443 | 5,511 |
2021 | 5,504 | 1,066 | 7,739 | 1,498 | 13,105 | 2,537 | 7,684 | 1,488 | 30,145 | 5,836 |
2022 | 5,834 | 1,128 | 8,202 | 1,587 | 13,890 | 2,687 | 8,144 | 1,575 | 31,950 | 6,180 |
2023 | 6,183 | 1,195 | 8,693 | 1,680 | 14,721 | 2,845 | 8,632 | 1,668 | 33,863 | 6,545 |
2024 | 6,553 | 1,266 | 9,214 | 1,779 | 15,603 | 3,013 | 9,148 | 1,767 | 35,890 | 6,931 |
2025 | 6,946 | 1,340 | 9,765 | 1,884 | 16,537 | 3,191 | 9,696 | 1,871 | 38,038 | 7,340 |
Table 3-13 Forecast Distribution and Trans Losses and Base Case Sales by Region
| Central Region | Northern Region | Southern Region | Western Region | Dhaka Region | |
Year | Sales (GWh) | Distrib. Loss (%) | Sales (GWh) | Distrib. Loss (%) | Sales (GWh) | Distrib. Loss (%) | Sales (GWh) | Distrib. Loss (%) | Sales (GWh) | Distrib. Loss (%) | Trans. Loss (%) |
2005 2006 2007 2008 2009 2010 2011 2012 2013 2014 2015 2016 2017 2018 2019 2020 2021 2022 2023 2024 2025 | 1,578 1,727 1,889 2,067 2,261 2,475 2,690 2,924 3,179 3,455 3,756 4,054 4,376 4,724 5,086 5,468 5,836 6,230 6,650 7,098 7,577 | 15.3% 14.9% 14.5% 14.1% 13.7% 13.3% 12.9% 12.5% 12.1% 11.7% 11.3% 10.9% 10.5% 10.1% 10.0% 10.0% 10.0% 10.0% 10.0% 10.0% 10.0% | 2,219 2,428 2,656 2,906 3,179 3,480 3,782 4,111 4,469 4,858 5,281 5,700 6,153 6,641 7,151 7,688 8,206 8,759 9,350 9,980 10,653 | 15.3% 14.9% 14.5% 14.1% 13.7% 13.3% 12.9% 12.5% 12.1% 11.7% 11.3% 10.9% 10.5% 10.1% 10.0% 10.0% 10.0% 10.0% 10.0% 10.0% 10.0% | 3,758 4,111 4,498 4,921 5,383 5,893 6,405 6,962 7,568 8,226 8,942 9,652 10,419 11,246 12,110 13,018 13,896 14,833 15,833 16,901 18,040 | 15.3% 14.9% 14.5% 14.1% 13.7% 13.3% 12.9% 12.5% 12.1% 11.7% 11.3% 10.9% 10.5% 10.1% 10.0% 10.0% 10.0% 10.0% 10.0% 10.0% 10.0% | 2,203 2,411 2,637 2,885 3,157 3,455 3,756 4,082 4,437 4,823 5,243 5,659 6,109 6,594 7,100 7,633 8,148 8,697 9,283 9,909 10,578 | 15.3% 14.9% 14.5% 14.1% 13.7% 13.3% 12.9% 12.5% 12.1% 11.7% 11.3% 10.9% 10.5% 10.1% 10.0% 10.0% 10.0% 10.0% 10.0% 10.0% 10.0% | 7,671 8,461 9,333 10,297 11,361 12,544 13,755 15,086 16,548 18,154 19,920 21,707 23,660 25,792 27,856 29,945 31,964 34,119 36,420 38,875 41,497 | 30.0% 28.5% 27.0% 25.5% 24.0% 22.5% 21.0% 19.5% 18.0% 16.5% 15.0% 13.5% 12.0% 10.5% 10.0% 10.0% 10.0% 10.0% 10.0% 10.0% 10.0% | 3.5% 3.5% 3.5% 3.5% 3.5% 3.4% 3.4% 3.3% 3.3% 3.2% 3.2% 3.1% 3.1% 3.0% 3.0% 3.0% 3.0% 3.0% 3.0% 3.0% 3.0% |
Average growth | 8.2% | | 8.2% | | 8.2% | | 8.2% | | 8.8% | |
3.4.1 Electricity Availability By 2020
The GOB’s goal of achieving electricity for all by 2020 is ambitious. As we understand it, this refers to having electricity available to every populated area. Not all potential customers will choose to connect to the system.
In its 2000 Master Plan, REB noted that by 2000 it had brought electricity service within the reach of about 30 million rural people, about 31% of the total rural population. Rural population comprised 75% of the total population of 130 million. It then had 2.85 million customers, peak demand of 973 MW, and energy demand of 2,650 GWH.
Its forecasts for 2020 were 9.31 million customers and 97 million rural people with electricity service within reach, which would be about 84% of the total rural population. Its forecast of peak demand for 2020 was 3,681 MW, with an energy demand of 13,060 GWH.
With 84% of the rural population within reach of electricity, the national coverage would be expected to be an even higher percentage.
The growth rates REB forecast for the periods 2000 –2020 and 2005 – 2020 were about 8% per year and equal to our Base Case forecasts over the same periods. Through 2004, REB has grown faster than its forecast, primarily because of transfers of large amounts of load from other utilities. National demand has grown at a steady 8% per year pace throughout that period, the transfers increasing REB’s growth rate while depressing the utilities that had load transferred to REB.
REB forecasts that 84% of the population in its area would have electricity service within reach by 2020. The urban areas should have an even higher percentage. REB’s forecast growth rate to achieve its 84% is nearly identical to our overall national growth rate.
Coverage of 84% of the rural population is less than coverage of all. Nevertheless, our forecast seems reasonably consistent with goal of achieving electricity for all by 2020.
3.5 COMPARISON OF FORECASTS
In this section, we compare our updated forecast with the forecast developed for the 1995 Power System Master Plan (1995 PSMP), the recent forecast developed as part of the current ADB Gas Development Project, and Case A of the recent forecast developed as part of the 2005 Gas Sector Master Plan. The consultants deem Case A to be their Base Case. The 1995 study developed a forecast only until year 2015 and the ADB Gas study listed values only for energy. The results are presented in Table 3-14 and Figure 3-5. All four forecasts are very close; the gas study would be even closer if the gross generation figures were reduced to correspond to the net values used in the other two studies. This confirms earlier observations that 1995 PSMP load forecast was very accurate, and also demonstrates that the calculations for natural gas use in the power sector in the ADB Gas study and in the 2005 Gas Sector Master Plan study are based on an electricity generation forecast similar to the Base Case of this study.
Table 3-14 Comparison of Forecasts
| Base Case | 1995 Power Sector Master Plan | ADB Gas Development. Project | 2005 Gas Sector Master Plan |
Fiscal Year | Net Gen- eration (GWh) | Net Peak Load (MW) | Net Gen- eration (GWh) | Net Peak Load (MW) | Gross Gen- eration (GWh) | Net Peak Load (MW) | Net Gen- eration (GWh) | Net Peak Load (MW) |
2005 | 21,964 | 4,308 | 22,823 | 4,342 | 24,161 | | 21,989 | 4,304 |
2006 | 23,945 | 4,693 | 24,662 | 4,692 | 26,108 | | 23,361 | 4,568 |
2007 | 26,106 | 5,112 | 26,651 | 5,070 | 28,214 | | 24,818 | 4,848 |
2008 | 28,461 | 5,569 | 28,804 | 5,480 | 30,492 | | 26,366 | 5,145 |
2009 | 31,028 | 6,066 | 31,133 | 5,923 | 32,959 | | 28,581 | 5,571 |
2010 | 33,828 | 6,608 | 33,654 | 6,403 | 35,628 | | 30,982 | 6,032 |
2011 | 36,622 | 7,148 | 36,300 | 6,906 | 38,428 | | 33,584 | 6,532 |
2012 | 39,647 | 7,732 | 39,157 | 7,450 | 41,452 | | 36,406 | 7,073 |
2013 | 42,922 | 8,364 | 42,243 | 8,037 | 44,720 | | 39,464 | 7,659 |
2014 | 46,467 | 9,047 | 45,578 | 8,671 | 48,250 | | 42,780 | 8,294 |
2015 | 50,306 | 9,786 | 49,180 | 9,357 | 52,064 | | 46,374 | 8,981 |
2016 | 54,079 | 10,512 | | | 56,229 | | 50,269 | 9,726 |
2017 | 58,135 | 11,291 | | | 60,727 | | 54,493 | 10,531 |
2018 | 62,496 | 12,128 | | | 65,586 | | 59,070 | 11,404 |
2019 | 67,183 | 13,027 | | | 70,832 | | 64,033 | 12,349 |
2020 | 72,222 | 13,993 | | | 76,499 | | 69,412 | 13,372 |
2021 | 77,092 | 14,924 | | | 80,324 | | 75,029 | 14,439 |
2022 | 82,290 | 15,917 | | | 84,340 | | 81,100 | 15,591 |
2023 | 87,839 | 16,977 | | | 88,557 | | 87,662 | 16,835 |
2024 | 93,761 | 18,107 | | | 92,985 | | 94,755 | 18,178 |
2025 | 100,083 | 19,312 | | | 97,634 | | 102,422 | 19,628 |
Figure 3-5 Comparison of Forecasts
3.6 SUBSTATION LOAD FORECAST
The demand forecasts presented thus far have addressed national and regional demand as determined by the demand measured at the high side of power plant main transformers. Transmission analysis requires forecasts of demand at the 132 KV buses of 132/33 KV substations. The total demand at these measuring points is less than the demand at power plant main transformers by the amount of transmission losses.
Based on our analysis of historical trends, we forecast that demand would grow at the same rate in each region as nationally. We did not constrain individual substations to grow at this rate. We forecast demand at each substation with the following procedure.
First we calculated equivalent national and regional total demand at transmission substations by adjusting demand at the high voltage side of power plant main transformers to account for transmission losses.
Based on an earlier forecast of overall demand in 2010, PGCB had developed a transmission expansion plan to 2010. This included many new substations that did not exist in 2005, and other facilities. In adding new substations, they split the load at overloaded existing substations. To determine the 2010 demand at each substation, they took into account their view of likely differences in growth rates of demand in different localities. For example, they forecast that northern Dhaka would grow faster than the other parts of Dhaka. These substation loads were then scaled so that the overall demand in each region equaled the regional demand forecast from Section 3.2.4, as adjusted for transmission losses. This produced a forecast of substation demand at 132 KV buses at each transmission substation expected to exist in 2010, including new substations.
From these regional distributions it was possible to calculate the fraction of total regional load demanded by each substation in 2010. We assumed that these regional load distributions would continue until 2025. Using our regional demand forecast for 2025, we calculated the demand at individual substations by multiplying total regional demand by the fraction determined for each substation. This generated the substation load forecast for 2025.
We conducted transmission analysis and planning using this distribution of loads, along with many other parameters. The product of these analyses was the least cost transmission system for 2025. We did not split substations to accommodate overloads. Rather, we added transformers and other components so that the substation demand could be served while maintaining planning criteria. As time draws nearer, new substations will have to be developed.
We developed substation load forecasts for 2020 and 2015 as we did for 2025. Based on our regional demand forecast for those years and the fractional distribution of demand for each substation, we calculated the pattern of transmission substation loads for each of those years.
Appendix A provides the substation load forecasts, in MW and MVAR, for each study year.
3.6.1 Reactive Demand
Our analysis to this point has dealt with active demand, as expressed in MW. However, for transmission analysis purposes reactive demand is also important. Accordingly we forecast
reactive demand, which is typically expressed in MVAR or power factor. Power factor does not in itself represent reactive demand, but can be used to calculate reactive demand if the active power in MW is known.
We reviewed data on power factor during the peak day (17 April) of fiscal 2005, including both the daytime peak and the evening peak. Data from more than 70 substations, many with several transformers for which separate data exists, showed that a substantial majority of power factors fell in the range 0.85 to 0.95 during the mid-day peak around noon and during the evening peak, the maximum of the day. Power factors below 0.80 occurred on only four transformers during the evening peak, and on nine during the daytime peak. Power factor is typically lower during the daytime peak because more of the load in the evening is cooking and lighting load that contributes little to reactive demand. The lower power factors during mid-day peaks are less important because demand and power flows are lower.
Based on this data and on discussions with BPDB and PGCB, this study used a power factor of 0.90 for demand at all substations in the transmission analysis study years of 2010, 2015, 2020, and 2025. This corresponds to an assumption that improvements in distribution system design, equipment, and tariff structure will improve power factors in the distribution systems by 2010 such that a power factor of 0.90 or more can be achieved at each substation.
3.7 COMPANY LOAD FORECAST
An additional factor complicates forecasting demand by company. Movement of parts of one organization’s service territory to another organization makes using historical demand data difficult. Future transfers seem certain, and BPDB is being restructured into several regional distribution companies. We developed a forecast by company based on the following assumptions:
- All demand is split among BPDB, DESA, DESCO, and REB.
- 2005 demand split is the same as 2004, after accounting for the assumed move of Tongi demand from DESA to DESCO.
- The shares of DESCO and REB increase steadily from 2005 – 2025 as the shares of BPDB and DESA decline.
− DESCO’s share grows from 11.1% in 2005 after Tongi transfer to 12% in 2025.
− REB’s share grows from 33.5% in 2005 to 35% in 2025.
− BPDB’s share declines from 32.4% in 2005 to 31% in 2025.
− DESA’s share declines from 22.9% in 2005 after Tongi transfer to 22% in 2025.
Table 3-15 presents the results. REB’s imports exclude 240 GWH from REB’s own generation.
Table 3-15 Demand Forecast by Company
| BPDB | DESA | DESCO | REB |
Year | Net Gen- eration (GWh) | Trans. Loss (%) | Imports (GWh) | Sales (GWh) | Imports (GWh) | Sales (GWh) | Imports (GWh) | Sales (GWh) | Imports (GWh) | Sales (GWh) |
2005 | 21,964 | 3.5% | 6,877 | 5,410 | 4,860 | 3,182 | 2,360 | 1,909 | 7,101 | 6,129 |
2006 | 23,945 | 3.5% | 7,481 | 5,941 | 5,288 | 3,548 | 2,583 | 2,105 | 7,758 | 6,715 |
2007 | 26,106 | 3.5% | 8,139 | 6,525 | 5,754 | 3,954 | 2,826 | 2,320 | 8,476 | 7,357 |
2008 | 28,461 | 3.5% | 8,854 | 7,165 | 6,261 | 4,404 | 3,092 | 2,558 | 9,260 | 8,060 |
2009 | 31,028 | 3.5% | 9,632 | 7,867 | 6,813 | 4,902 | 3,384 | 2,819 | 10,117 | 8,830 |
2010 | 33,828 | 3.4% | 10,484 | 8,641 | 7,417 | 5,457 | 3,704 | 3,109 | 11,059 | 9,679 |
2011 | 36,622 | 3.4% | 11,332 | 9,425 | 8,018 | 6,029 | 4,027 | 3,404 | 12,004 | 10,535 |
2012 | 39,647 | 3.3% | 12,248 | 10,279 | 8,668 | 6,658 | 4,378 | 3,728 | 13,030 | 11,467 |
2013 | 42,922 | 3.3% | 13,238 | 11,209 | 9,370 | 7,350 | 4,759 | 4,081 | 14,143 | 12,481 |
2014 | 46,467 | 3.2% | 14,308 | 12,222 | 10,130 | 8,109 | 5,173 | 4,468 | 15,351 | 13,585 |
2015 | 50,306 | 3.2% | 15,465 | 13,326 | 10,951 | 8,944 | 5,623 | 4,891 | 16,663 | 14,786 |
2016 | 54,079 | 3.1% | 16,598 | 14,426 | 11,755 | 9,791 | 6,070 | 5,317 | 17,959 | 15,980 |
2017 | 58,135 | 3.1% | 17,813 | 15,617 | 12,618 | 10,715 | 6,552 | 5,779 | 19,356 | 17,270 |
2018 | 62,496 | 3.0% | 19,117 | 16,904 | 13,545 | 11,721 | 7,072 | 6,281 | 20,862 | 18,665 |
2019 | 67,183 | 3.0% | 20,517 | 18,295 | 14,540 | 12,818 | 7,633 | 6,826 | 22,485 | 20,171 |
2020 | 72,222 | 3.0% | 22,008 | 19,790 | 15,600 | 14,005 | 8,234 | 7,414 | 24,222 | 21,788 |
2021 | 77,092 | 3.0% | 23,441 | 21,097 | 16,618 | 14,957 | 8,820 | 7,938 | 25,909 | 23,318 |
2022 | 82,290 | 3.0% | 24,967 | 22,470 | 17,704 | 15,933 | 9,448 | 8,503 | 27,713 | 24,941 |
2023 | 87,839 | 3.0% | 26,591 | 23,932 | 18,860 | 16,974 | 10,120 | 9,108 | 29,642 | 26,678 |
2024 | 93,761 | 3.0% | 28,322 | 25,490 | 20,091 | 18,082 | 10,840 | 9,756 | 31,706 | 28,535 |
2025 | 100,083 | 3.0% | 30,165 | 27,149 | 21,403 | 19,263 | 11,611 | 10,450 | 33,913 | 30,522 |
Section 4 Fuel Supply
4.1 INTRODUCTION
Presently, natural gas is the only significant source of commercial energy in Bangladesh. About 85% of the power generation capacity in the country is gas based, 10% is imported oil based and 5% hydro. About 90% of electrical energy is generated by natural gas. However, Barapukuria Coal Mine in the north-west region of Bangladesh is under development and it will supply coal to BPDB's first coal based 2x125 MW power plant at Barapukuria (now under construction) by FY2006. There are also very good prospects of extraction of coal from a nearby coal deposit at Phulbari. Asia Energy Corporation Bangladesh Ltd. (AECB), fully owned by Asia Energy PLC of UK is currently carrying out a Definitive Feasibility Study on the Phulbari coal project.
Bangladesh Oil, Gas and Mineral Corporation (BOGMC, also known as Petrobangla), operates as a statutory body created by the Ordinance of 1985. The Corporation is under the administrative control of the Energy and Mineral Resources Division of the Ministry of Power, Energy and Mineral Resources. The major functions and responsibilities of Petrobangla include:
- Exploration and development of oil, gas and mineral resources in the country.
- Coordination, planning and supervision of the activities of its subordinate companies.
- Overall control and coordination of the production, transmission and marketing of gas, condensate, oil and mineral resources produced in the country.
- Implementation of production sharing contracts with International Oil Companies for exploration and development of oil and gas.
Currently there are eleven companies operating under Petrobangla, responsible for oil and gas exploration, production, transmission, distribution, and development and marketing of coal and hard rock. One of these companies is Bangladesh Petroleum Exploration and Production Company Limited (BAPEX).
This section provides the present status and future prospects of the country's gas and coal resources, applicable for the planning of the power sector.
4.2 EXISTING GAS SUPPLY
4.2.1 Present Reserves
Total proven and probable recoverable gas reserves in Bangladesh from 22 fields (21 in East Zone and 1 in West Zone) is about 20 trillion cubic feet (TCF), of which about 5 TCF has so far been consumed. The net recoverable volume of gas is about 15 TCF. All the 12 producing gas fields are in the East Zone of the country. Table 4-1 provides the reserve quantity for each of the gas fields.
Table 4-1 Natural Gas Reserves of Bangladesh
FIELD | Recoverable = Proven + Probable, BSCF | Cumulative Production, BSCF | Net Remaining Recoverable, BSCF |
A. PRODUCING | | |
1. | Bakhrabad | 1002 | 624 | 378 |
2. | Beanibazar | 170 | 23 | 147 |
3. | Habiganj | 3854 | 1089 | 2765 |
4. | Jalalabad | 879 | 158 | 721 |
5. | Kailashtila | 1931 | 317 | 1614 |
6. | Meghna | 111 | 32 | 79 |
7. | Narshingdi | 77 | 47 | 30 |
8. | Rashidpur | 1401 | 307 | 1094 |
9. | Sangu | 734 | 247 | 490 |
10. | Saldanadi | 140 | 32 | 108 |
11. | Sylhet | 479 | 172 | 307 |
12. | Titas | 5110 | 2179 | 2931 |
B. NOT IN PRODUCTION | | |
13. | Begumganj | 32 | 0 | 32 |
14. | Bibiyana | 2202 | 0 | 2202 |
15. | Fenchuganj | 283 | 0 | 283 |
16. | Kutubdia | 603 | 0 | 603 |
17. | Simutang | 122 | 0 | 122 |
18. | Shahbazpur | 465 | 0 | 465 |
19. | Maulavibazar | 350 | 0 | 350 |
C. PRODUCTION SUSPENDED | | |
20. | Chattak | 332 | 26.5 | 305.5 |
21. | Feni | 116 | 39.51 | 76.49 |
22. | Kamta | 27 | 21.1 | 5.9 |
TOTAL (TCF) | 20.42 | 5.31 | 15.11 |
Source : Petrobangla Annual Report 2003 |
4.2.2 Gas Production
Present gas production capacity (as of November 2004) is 1,365 MMCFD (million cubic feet per day). Production Augmentation Projects planned for implementation during 2005-2010 will provide an additional 1,028 MMCFD of gas. Gas production capacity (as of June 2003) and actual production during FY 2003 of the various fields are given in Table 4-2.
Table 4-2 Natural Gas Production (FY2003)
Field | Capacity, Mmcfd | Production, Yearly Bscf | Production, Average Daily Mmcfd |
Titas | 375 | 134 | 368 |
Habiganj | 263 | 95 | 260 |
Bakhrabad | 35 | 13 | 35 |
Narshingdi | 17 | 6 | 15 |
Meghna | 8 | 2 | 6 |
Sub-Total | 698 | 250 | 684 |
Sylhet | 5 | 2 | 5 |
Kailashtila | 85 | 30 | 81 |
Rashidpur | 112 | 38 | 105 |
Beanibazar | 35 | 8 | 21 |
Sub-Total | 237 | 78 | 212 |
Saldanadi | 17 | 6 | 17 |
Sangu | 172 | 52 | 142 |
Jalalabad | 140 | 36 | 98 |
Sub-Total | 329 | 94 | 257 |
TOTAL | 1,264 | 421 | 1,154 |
4.2.3 Gas Transmission System
There are 1,832 KM of gas transmission pipelines in the country, most of which are located in East Zone. The diameters of the major pipelines are 24 and 30 inches. Maximum flow capacity of the major pipelines ranges from 175 MMCFD to 360 MMCFD. Other gas transmission projects are at various stages of implementation.
4.2.4 Gas Supply to West Zone
The major river Jamuna was an obstacle to the transmission of gas from East Zone to the West Zone. This obstacle was removed when the construction of the 4.8 KM long Jamuna Bridge was completed in 1998 and a gas transmission pipeline was laid on the Bridge. This gas transmission pipeline, named Elenga-Nalka, connects Nalka on the west side of the bridge with Elenga on the east. The size of the pipeline is 30 inch on the bridge and 24 inch on either side of the bridge. The total length of the Elenga-Nalka pipeline is 37 KM and the maximum flow capacity is 280 MMCFD. Subsequently this pipeline was extended from Nalka to Baghabari, where gas is supplied to the Baghabari power station of BPDB (71+100 MW) and Westmont IPP (130 MW). The length of Nalka-Baghabari pipeline is 36 KM, its size is 20 inch with a maximum flow capacity of 175 MMCFD. Plans are to extend the gas transmission network in the West Zone further to the north- west and south- west of the country.
4.2.5 Gas Consumption
The total gas consumption was about 400 BSCF against a production of 421 BSCF during FY2003. The daily gas consumption was 1,340 MMCF as of November 2004, out of which the power sector accounted for 40%. Table 4-3 provides gas consumption by major consumers during FY2003.
Table 4-3 Gas Consumption in FY2003
Category of Consumers | Consumption (BSCF) |
Power | 190.54 |
Fertilizer | 95.89 |
Industry | 63.75 |
Domestic | 44.80 |
Commercial | 4.36 |
Tea Estates | 0.744 |
Brick Fields | 0.527 |
4.3 PRODUCTION AUGMENTATION PROJECTS
Petrobangla has taken up a number of gas production augmentation projects for implementation during FY2005-2010. The resultant additional gas supply would be 1,028 MMCFD. Table 4-4 provides the list of the projects.
Table 4-4 Production Augmentation Projects (FY2005-2010)
Fields | Programs | Imple- menting Agency | Imple- mentation Time | Additional Gas, MMCFD |
Fenchuganj | Drilling of well #3 & process plants | Bapex | Dec. 2004 | 30 |
Sylhet | Workover, well #7 | CGFL | Feb. 2005 | 15 |
Moulvibazar | Production starts | Unocal | Mar 2005 | 70 |
Moulvibazar | Drilling 2 wells | Unocal | Apr 2005 | 70 |
Kailastila | Workover, well # 3 & 4 | SGFL | Dec 2005 | 25 |
Kailastila | Drilling well # 5 | SGFL | Dec 2005 | 25 |
Titas | Drilling well # 15 | BGFCL | Dec 2005 | 25 |
Titas | Drilling well # 16 | BGFCL | June 2006 | 25 |
Kailastila | Drilling well # 6 | BGFCL | Dec 2006 | 25 |
Bibiyana | Drilling 6 well | Unocal | Dec 2006 | 250 |
Habiganj | Drill 1 well | BGFCL | 2006-07 | 30 |
Narsingdi | Drill 1 well | BGFCL | 2006-07 | 10 |
Bibiyana | Drilling 3 well | Unocal | Dec 2007 | 125 |
Shahbajpur (Bhola) West Zone | Drill 1 well + plant | Bapex | 2007-08 | 40 |
Bibiyana | Drilling 3 well | Unocal | Dec 2008 | 118 |
Kailastila | Drill 2 wells | SGFL | 2008-09 | 50 |
Bakhrabad | Drill 2 wells | BGFCL | 2009-10 | 20 |
Habiganj | Drill 1 well | BGFCL | 2009-10 | 30 |
Titas | Drill 2 wells | BGFCL | 2009-10 | 50 |
TOTAL | | | | 1,028 |
Source: Petrobangla |
4.4 FUTURE GAS SUPPLY PROSPECTS
4.4.1 Projected Gas Demand
Table 4-5 gives the projected maximum gas demand of major consumers during the period FY2005-2010. Note that the demand of the power sector in about 50% of total demand.
Table 4-5 Projected Maximum Gas Demand (FY2005-2010)
Sector | FY2005 | FY2006 | FY2007 | FY2008 | FY2009 | FY2010 |
Power | 775 | 840 | 871 | 942 | 1,024 | 1,024 |
Fertilizer | 287 | 287 | 287 | 287 | 287 | 287 |
Others | 568 | 653 | 732 | 819 | 918 | 918 |
Total | 1,630 | 1,780 | 1,890 | 2,048 | 2,229 | 2,229 |
Overall Demand | 1,520 | 1,691 | 1,796 | 1,843 | 2,006 | 2,168 |
Source: Petrobangla |
4.4.2 Gas Requirement for Power
The base case (Sufficient Gas Scenario, Reference Load Forecast) WASP-IV analysis identified the least cost option for generation capacity addition during the planning period (2005-2025) to meet electricity demand reliably. The analysis also identified the fuel requirement for power generation for the 20-year period. Table 4-6 gives the annual energy generation and corresponding gas requirement. The values shown are reasonably consistent with the Petrobangla forecast shown in Table 4-5. The total quantity of gas required during the next 20 years is about 9.5 TCF. Table 4-6 also shows that about 10 million tons of coal would be required. This quantity of coal will be required for operation of the Barapukuria 2x125 MW power plant that will commence operation by November 2005.
Table 4-6 Gas and Coal Requirement During 2005-2025 (Sufficient Gas Scenario)
Year | Peak Load, MW | Energy, GWH | Gas Requirement, BSCF | Coal Requirement, Million Tons |
2005 | 4,308 | 22,077 | 224.9 | 0 |
2006 | 4,693 | 24,050 | 230.7 | 0.326 |
2007 | 5,112 | 26,197 | 254.7 | 0.644 |
2008 | 5,569 | 28,539 | 277.4 | 0.635 |
2009 | 6,066 | 31,086 | 303.7 | 0.569 |
2010 | 6,608 | 33,864 | 318.3 | 0.530 |
2011 | 7,148 | 36,631 | 343.5 | 0.523 |
2012 | 7,732 | 39,624 | 367.1 | 0.507 |
2013 | 8,364 | 42,863 | 393.1 | 0.509 |
2014 | 9,047 | 46,363 | 421.6 | 0.500 |
2015 | 9,786 | 50,150 | 456.8 | 0.498 |
2016 | 10,512 | 53,871 | 487.1 | 0.495 |
2017 | 11,291 | 57,863 | 534.1 | 0.497 |
2018 | 12,128 | 62,152 | 572.3 | 0.495 |
2019 | 13,027 | 66,759 | 543.5 | 0.525 |
2020 | 13,993 | 71,710 | 578.6 | 0.511 |
2021 | 14,924 | 76,481 | 607.4 | 0.518 |
2022 | 15,917 | 81,570 | 640.7 | 0.507 |
2023 | 16,977 | 87,002 | 677.9 | 0.502 |
2024 | 18,107 | 92,793 | 717.0 | 0.498 |
2025 | 19,312 | 98,968 | 762.3 | 0.502 |
TOTAL | | | 9,509 | 10.29 |
4.4.3 Gas Supply
The National Energy Policy of the Government of Bangladesh provides an allocation of 50% of total reserve and Maximum Daily Production of natural gas for power generation. As Table 4-6 shows, the total requirement of gas for power generation during 2005-2025 is 9.5 TCF. This is about 63% of present remaining reserve of 15 TCF. Therefore, more reserve would be required to be discovered to raise the reserve level to about 20 TCF to satisfy the requirement of the least cost power development plan. The probability of increase in the gas reserve is discussed below.
4.4.3.1 Exploration Activity
Bangladesh formulated a new Petroleum Policy in 1993 for exploitation of petroleum resources through systematic evaluation, exploration, and development. In pursuance of this Petroleum Policy, GOB through Petrobangla invited International Oil Companies (IOCs) to explore for hydrocarbons in Bangladesh under attractive terms and conditions. Bangladesh is divided into 23 Blocks for exploration and development. There have been two rounds of bidding and the responses of the IOCs have been very encouraging, with highly satisfactory results. 17 Blocks, including 6 off-shore Blocks were opened up for exploration. Currently, blocks awarded under two round of bidding are operational. Under the 1993/94 awards, blocks 12, 13, and 14 (East Zone) were awarded to Occidental (now Unocal Bangladesh), blocks 15 and 16 (in East Zone) to Cairn Energy (now operated by Shell Bangladesh), blocks 17 and 18 to Rexwood/Okland (now operated by Tullow Oil) and block 22 to UMC (now operated by Ocean Energy). Under Production Sharing Contracts (PSCs), the respective operators have conducted varying amount of exploration and development works.
Under the second bidding round, four blocks have been awarded. Unocal Bangladesh was awarded block 7, Shell/Cairn Joint Venture was awarded blocks 5 and 10 with 10% carried stake for BAPEX, and Tullow/Chevron/Texaco JV was awarded block 9 with 10% carried stake for BAPEX. Among these blocks, seismic survey is planned in block 10, while in block 9 major seismic surveys including a 3-dimensional survey have been completed, followed by a three well drilling campaign currently underway. Between 1972 and 2004, a total of 39 exploratory wells were drilled by national and international companies, which resulted in the discovery of 13 gas fields.
From the above discussion, it appears that there will almost certainly be additions to existing reserves as exploration proceeds. The success rate of previous exploration was high (one in three) and the IOCs have shown keen interest in exploration activities. Remaining recoverable reserve of gas was 10 TCF in 1995, and increased to 15 TCF by 2005 despite the usage of gas at an accelerated rate during this period. Thus, it can be concluded that availability of 10 TCF of gas for power generation during the next 20 years is highly probable.
4.5 COAL RESOURCES
4.5.1 National Estimates
The Geological Survey of Bangladesh (GSB) is responsible for locating coal resources present in Bangladesh, but public sector development of any coal resources is undertaken by Petrobangla. Coal resources estimated at 2,514 million tons have been discovered in closely
spaced locations in the North-West of the country. Table 4-7 below gives the locations of the deposits, proven reserves, and status of development.
The coal production and reserves of the Barapukuria Coal Mine and the Phulbari Coal Project mines would be adequate to supply coal for power generation capacity of 2,750 MW or more. However, for the Base Case Scenario, the only coal units included are the two 125 MW units at Barapukuria. In that case, the development of the Phulbari Coal Project as outlined in Table 4-8 below would have to rely on selling to other sources of demand.
Table 4-7 Estimated Coal Reserves
Location / Field | Year of Discovery | Drilled Well | Depth (Meter) | Proven Reserve (Million Tonnes) | Field Status |
Barapukuria | 1985-87 | 31 | 118-509 | 390 | Under development |
Khalaspir Rangpur | 1989-90 | 4 | 257-483 | 685 | Awarded to China Bangladesh Consortium |
Phulbari Dinajpur | 1997 | 1 | 150-240 | 386 | Awarded to Asia Energy Co |
Jamalganj Joypurhat | 1962 | 10 | 640-1158 | 1053 | |
Dighipara Dinajpur | 1994-95 | 3 | 328-407 | Not yet estimated | |
Source : Petrobangla |
In the Limited Gas Scenario, we assume that 4,000 MW of new power plant capacity is developed using domestic coal. In order to achieve that goal, development beyond that planned for Barapukuria and Phulbari would be needed. The reserves listed in Table 4-7 seem to support domestic coal supplies for 4,000 MW of power plant capacity, or possibly even more, as a reasonable expectation.
4.5.2 Barapukuria Coal Mine
The development of Barapukuria Coal Mine by Petrobangla is nearing completion. Full production will commence by the November 2005 with an estimated annual production of 1.0 million tonnes for 30 years. Two 125 MW power plant units are under construction and are all the generation planned for that site, but the estimated annual production could supply at least three such units. A second phase of mine development could further expand coal supply and serve more power generating units. The Barapukuria coal is classified as high volatile B bituminous (ASTM). The Barapukuria coal is of good quality, with heat content of about 25,000 GJ/kg (10,500 Btu/lb) and a sulfur content of about 0.5%. Compared with many coals on the subcontinent, these are very good figures.
4.5.3 Phulbari Coal Project
The location is in the district of Dinajpur near Barapukuria. The Phulbari Exploration Licenses for the project have been awarded to Asia Energy Corporation, Bangladesh Ltd. fully owned by Asia Energy PLC incorporated in UK. The Company is carrying out a Definitive Feasibility Study for developing and operating an open cut mine. The Feasibility Study is scheduled to be completed by 2005-06. The in-situ coal reserve is estimated to be 430 million tonnes. The project timetable is provided in Table 4-8. The potential quality of coal at Phulbari is outstanding. Phulbari’s high volatile A and B bituminous coal is a low ash, low sulfur product, highly suitable for export markets and power generation. The coal
production and reserve would be adequate to supply coal for a power plant of about 2,500 MW.
Table 4-8 Phulbari Mine Development Timetable
Period | Year | Activity | Coal Production, Million Tonnes |
Mine Development Years 1-2 Year-3 | 2004-2005 2006 | Project feasibility assessment Mining approval, mine development and commencing groundwater dewatering. | |
Coal Production | | | |
Year 4 | 2007 | Pre-stripping, overburden removal and coal extraction. | 1.5 |
Year 5 | 2008 | Pre-stripping, overburden removal and coal extraction. | 6 |
Year 6 | 2009 | Pre-stripping, overburden removal and coal extraction. | 9 |
Years 7 – 8 | 2010-2011 | Pre-stripping, overburden removal and coal extraction. | 12 |
Year 9 | 2012 | Pre-stripping, overburden removal and coal extraction. | 14 |
Year 9 - 34 | 2013-2037 | Pre-stripping, overburden removal and coal extraction. | 15 |
Source: Asia Energy Corporation |
4.6 PRICE OF FUELS TO 2025
The existing fuel-burning plants use natural gas, furnace oil, and diesel oil. A domestic coal- fired plant is nearly complete. Options for future plants include both domestic and imported coal-fired steam plants. Accordingly, we prepared price forecasts for these fuels.
4.6.1 Historical Prices
Table 4-9 shows historical prices for common power plant fuels in Bangladesh since 1991. It demonstrates that the GOB has maintained stable prices in US$ over that period. The prices shown are those that apply for purchases by BPDB from Petrobangla. All the fuels are liquid petroleum products and nearly entirely imported, except the natural gas that is Bangladesh’s main domestic fuel resource.
Table 4-9 Historical Fuel Prices
Fuel | Kerosine Oil | High Speed Diesel Oil | Light Diesel Oil | Furnace Oil | Natural Gas | Exchange Rate, Taka/Dollar |
Effective Date | Taka/ liter | US$/ liter | Taka/ liter | US$/ liter | Taka/ liter | US$/ liter | Taka/ liter | US$/ liter | Taka/ 1000 ft^3 | US$/ 1000 ft^3 |
1-Jul-91 | 13.71 | 0.35 | 13.71 | 0.35 | 12.19 | 0.31 | 7.41 | 0.19 | 39.08 | 1.00 | 38.95 |
1-May-92 | 13.71 | 0.35 | 13.71 | 0.35 | 12.19 | 0.31 | 7.41 | 0.19 | 43.05 | 1.11 | 38.95 |
1-Jun-92 | 13.41 | 0.34 | 13.41 | 0.34 | 11.89 | 0.31 | 7.11 | 0.18 | 43.05 | 1.11 | 38.95 |
1-Apr-94 | 13.41 | 0.33 | 13.41 | 0.33 | 11.89 | 0.30 | 7.11 | 0.18 | 47.58 | 1.19 | 40.15 |
1-Jul-95 | 12.41 | 0.30 | 12.41 | 0.30 | 11.89 | 0.29 | 4.51 | 0.11 | 47.58 | 1.14 | 41.65 |
1-Sep-98 | 12.66 | 0.26 | 12.63 | 0.26 | 13.40 | 0.28 | 5.00 | 0.10 | 47.58 | 0.98 | 48.35 |
1-Dec-98 | 12.66 | 0.26 | 12.63 | 0.26 | 13.40 | 0.28 | 5.00 | 0.10 | 54.66 | 1.13 | 48.35 |
16-Aug-00 | 15.17 | 0.27 | 15.09 | 0.27 | 16.22 | 0.29 | 6.50 | 0.12 | 62.87 | 1.11 | 56.50 |
27-Dec-01 | 16.67 | 0.29 | 16.59 | 0.29 | 17.60 | 0.31 | 12.50 | 0.22 | 62.87 | 1.10 | 57.40 |
6-Jan-03 | 16.67 | 0.29 | 16.59 | 0.29 | 17.60 | 0.31 | 12.50 | 0.22 | 65.99 | 1.15 | 57.40 |
8-Jun-04 | 16.83 | 0.29 | 19.83 | 0.35 | 19.71 | 0.34 | 10.00 | 0.17 | 70.00 | 1.22 | 57.40 |
6-Aug-04 | 16.83 | 0.28 | 19.83 | 0.33 | 19.71 | 0.33 | 12.00 | 0.20 | 70.00 | 1.17 | 60.00 |
Current Price | 22.52 | 0.36 | 22.37 | 0.36 | 22.01 | 0.36 | 12.00 | 0.19 | 73.91 | 1.19 | 62.00 |
4.6.2 Forecast Prices
4.6.2.1 Recommended Pricing Approach
The objective for establishing prices for power plant fuels is to support decision-making in the best interest of Bangladesh. Thus, rather than consider only BPDB and other power plant fuel buyers, we consider the impact of pricing on the country as a whole. Table 4-9 prices for the liquid fuels are reasonably close to world market prices. Natural gas is more difficult to move in international trade, making it harder to establish an international benchmark.
Natural gas will be by far the dominant fuel in the Base Case, so it is especially important to use prices for natural gas that serve Bangladesh’s long-term interests. Pricing that incorporates subsidies or ignores international markets risks negatively affecting decision- making.
Petrobangla has a clear monopoly on the market for the supply of power plant fuels. One alternative for pricing in monopoly situations is to base prices on the cost of providing the services and products. This is the best choice in many cases and appears to be the approach taken in Bangladesh. The historical fuel prices in Table 4-9 are reasonably consistent with our understanding of what Petrobangla’s costs may have been, based on world market prices for the liquid products listed plus some margin for its costs other than product acquisition.
The price for natural gas is reasonably consistent with our understanding of what Petrobangla’s costs for supplying natural gas to power plants may have been.
Market prices, such as usually prevail in non-monopoly situations, are viewed as superior to regulated prices because of the incentives they provide. However, they can’t be used in monopoly situations because there is no real market.
Another approach in monopoly situations is to use “opportunity costs” as a surrogate for market prices. An opportunity cost is the market-based cost of an alternative to the product for which the price is being used. The use of opportunity costs can offer better incentives and improve decision-making. However, depending on their application that can create their own distortions and lead to windfalls or the need for subsidies because the price is different than the cost.
An opportunity price approach for gas was used in the development of the 1995 Master Plan, which linked the gas price used in analysis to the forecast cost of fuel oil. As we understand it, the pricing of gas under some suppliers’ contracts with Petrobangla has some similarities to this approach. The “profit” between the suppliers’ costs and what could be characterized as an opportunity cost (calculated in a manner similar to both the 1995 Master Plan approach and our approach) is shared between the supplier and the GOB according to formula.
Several reasons argue for using an opportunity cost approach for pricing gas at least in our analysis.
- Gas can and does replace higher-cost liquid fuels in other applications in Bangladesh, such as transport. Exhausting the resource rapidly prevents its longer-term use for such high-value applications.
- As gas demand grows, future development of gas fields is likely to be more costly than what has gone before. Without pricing that encourages development, shortages may occur.
- As Bangladesh develops economically, something resembling a market for gas may develop. This may include more sources of end-user demand for gas, and international trade in natural gas. Worldwide experience suggests that gas prices in market situations tend to approach parity with some liquid fuel prices. We understand that some of Petrobangla’s Production Sharing Contracts use 75% of a fuel oil benchmark as the key part of the calculation of price.
For our use we are not attempting to determine the price that Petrobangla should charge BPDB. Rather, we are determining what price to use in our analysis. The results of the resource planning study will establish which new generating resources should be added. The results are affected by the price of gas. However, the price charged by Petrobangla is a separate issue and a policy choice for the GOB. Considerations in making such a choice might include the points bulleted above, and the desire to keep gas tariffs and electricity tariffs more affordable for consumers.
Based on our analysis of the issues discussed above, we chose the following pricing approach:
- Forecast market prices for all fuels except natural gas.
- Opportunity cost prices for natural gas, based on 75% of estimated world fuel oil prices.
4.6.3 Determining the Forecast Prices
The United States’ Energy Information Administration (EIA) produces yearly forecasts of the world prices of various fuels. We used the Reference Case in their Annual Energy Outlook 2005 as our source. They characterize the Reference Case for world oil price as follows:
“Reference World Oil Price Case. In the reference case, the assumption is that he OPEC members will continue to demonstrate a disciplined production approach that reflects a strategy of price defense in which the larger producers are willing to increase or decrease production levels to maintain fairly stable prices (in real dollar terms) to discourage the development of alternative crude supplies or energy sources, allow for continued robust worldwide economic growth, and maintain compliance with quotas, particularly by smaller OPEC producers. It is also assumed that OPEC producers will achieve sufficient oil revenues to expand production capacity enough to keep prices in a range of $27 to $30 per barrel in 2003 dollars, near the high end of the current OPEC price target a. Their current level of proven reserves (870 billion barrels) is sufficient to meet the implied production levels.
In the medium term, there is enough resource potential in non-OPEC countries to allow non- OPEC production to continue growing. Over the longer term, it is estimated that it will be harder for non-OPEC countries to continue to increase production. Assuming reference case prices, the search for alternatives and unconventional liquids will be limited, while demand will continue to grow. Therefore, OPEC members will have to make up the production difference [referenced to figure 132 in their report]. To satisfy the remaining global demand for oil at the given reference case price, OPEC production will have to increase from 30.6 million barrels per day to 55.1 million barrels per day, an average annual increase in production of 2.7%. This is projected to result in an increase in OPEC’s market share from 39% in 2003 to 46% in 2025, as cheaper sources of non-OPEC oil are depleted.
The reasoning behind the assumed prices and production patterns in the reference case can be questioned. If OPEC members have sufficient market power and cohesiveness to set world prices, why would they not try to set higher oil prices? If OPEC comprised a group of producer countries with similar oil reserves, resource depletion time horizons, geopolitical concerns, and no fear of alternatives to oil at higher prices, then a more limited production strategy that maximizes economic profits in the short to medium term would appear more plausible. In the absence of these conditions, however, and given the difficulty of enforcing tight production goals to limit output, a reasonable strategy is to maintain stable prices that discourage oil alternatives while limiting the risk that member countries will exceed their quotas.
Another issue is whether OPEC members will be able to finance the investments needed to expand their output as projected in the reference case. While some OPEC producer countries are currently closed to foreign involvement in the exploration and development of oil resources, it is expected that hey will be able to attract foreign capital, if needed, while retaining sovereignty over their energy resources. The markets for financial capital have provided sufficient resources in similar situation in the past, especially when there are strong incentives from both the demand and supply sides. The current experience of China, which did not attract much financial capital in the past, is an example of what can happen with the appropriate economic incentives or when the motivations are strong. Other historical examples include the flow of foreign capital to Latin America in the 1980s and East Asia in the 1990s.
There are also factors that may encourage countries in the Middle East to open up their energy sectors to foreign participation in one form or another. For example, Saudi Arabia, for some time now, has been lobbying to gain admission to the World Trade Organization. One of the conditions that Saudi Arabia needs to fulfill to gain entry is to open up its economy, especially its financial markets. The opening up of the United Arab Emirates to foreign financial capital and its creation of an export trade zone provide another example of how the economic environment can change.”
The Annual Energy Report 2005 also forecasts that US coal prices for exports, which could be the source for imported coal for Bangladesh and in any event must compete in the world market, are not expected to rise significantly. Increasing labor productivity permitted prices to decline by 4.9% per year from 1990 to 1999, but price increases are expected in 2005.
Competition from other fuel resources, slowing demand, and a shift in production to lower cost Western mines, and increasing labor productivity (at a lower rate than in the 1990s) contribute to modest price declines from 2005 to 2010, then flat prices through 2020, and slowly increasing prices from 2020 to 2025 and the price of competing fuels rises and demand grows more rapidly.
Our fuel price forecasts use basic data from the Annual Energy Outlook 2005 as the starting point. Specifically, we use the reference case crude oil price forecast and the reference case export coal price. EIA characterizes its forecasts as being of world market prices, in other words not specific to just the US. Both coal and especially oil are traded extensively in world markets. Thus prices in any open market situation should relate to their world market prices. The following steps produce the forecasts used in analysis:
Liquid Fuels and Natural Gas
- Tabulate the reference case forecast in 2003 dollars per barrel over the period 2005 to 2025.
- Convert to 2005 dollars to account for 3% inflation in each year 2004 and 2005.
- Convert to 2005 dollars per GJ.
- Calculate fuel oil price in dollars per GJ based on 75% of the price of crude oil. This is based on historical data for the ratio of fuel oil price to crude oil price.
- Calculate high sulfur diesel price in dollars per GJ based on 115% of the price of crude oil. This is based on historical data for the ratio of high sulfur diesel price to crude oil price.
- Calculate natural gas price in dollars per GJ (lower heating value basis) based on 75% of the price of fuel oil.
- Calculate levelized prices over 2005 – 2025 based on a 12% discount rate. WASP can accept only levelized prices, not yearly prices.
- Convert the levelized prices in $/GJ to $/million KCAL for input to WASP.
Imported Coal
- Tabulate the reference case forecast in 2003 dollars per short ton over the period 2005 to 2025.
- Convert to 2005 dollars to account for 3% inflation in each year 2004 and 2005.
- Add US$12.60 per short ton for coal transport and handling.
- Convert to dollars per GJ.
- Calculated levelized prices over 2005 – 2025 based on a 12% discount rate.
- Convert the levelized prices in $/GJ to $/million KCAL for input to WASP.
Domestic Coal
- Calculate domestic fuel price per short ton based on 80% of the price of imported coal including transport.
- Convert to dollars per GJ.
- Calculated levelized prices over 2005 – 2025 based on a 12% discount rate.
- Convert the levelized prices in $/GJ to $/million KCAL for input to WASP.
A market for domestic coal may develop in Bangladesh, given that several fields have been identified and more are possible. However, no realistic market exists today, so we must estimate prices using another approach. The key factor in the bullet list above is establishing the domestic fuel price at 80% of the price of imported coal, on a $/ton basis. The following concepts led us to this approach.
- Because coal is a world market product, domestic coal will have to compete with imported coal to supply power plants. Unless it is subsidized or protected from competition, it should cost no more than imported coal.
- If coal could be produced for much less than 80% as much as the cost of imported coal, it might well be economic to export it rather than use it domestically. In other words the world market price would influence the price of domestic coal regardless of its cost of production, unless the coal facility were required to subsidize other activities.
- The cost of coal, and to some extent its price, will vary substantially among mines, so no single price can be accurate for all mines. We understand that the Phulbari Coal Project may be able to supply its coal for less than the estimated forecast cost of imported coal, whereas the cost of coal from the Barapukuria Coal Mine may be higher.
Table 4-10 provides the resulting fuel prices used in the analysis.
Section 4 Fuel Supply
Table 4-10 Fuel Price Forecast
Section 5 Generation and Transmission Expansion Options and Sites
5.1 GENERATION OPTIONS
Fuel availability and cost drives the selection of generation options. Bangladesh has substantial proven reserves of natural gas and some proven reserves of coal. Both are available at reasonable cost. There is reason to expect that more natural gas and coal reserves will be discovered as exploration continues.
Bangladesh’s limited hydro potential will have been completely developed when the 100 MW extension of the existing Karnafuli plant is completed. Thus additional hydro capacity is not a feasible option for additional units.
The focus of our generation options will be on plants that can use natural gas or coal economically. We also provide data on nuclear, and touch briefly on generation based on renewable resources, whose capital costs and other considerations make them impractical compared to the natural gas and coal options.
5.1.1 Generation Technology Options
5.1.1.1 Steam Turbine
Steam turbine technology has provided much of world's base generating capacity since the beginnings of the industrial era. The technology has significantly improved over the past decades with respect to reliability, availability, and performance. The size of a single steam turbine unit has progressed to about 800-1,000 MW. High steam temperature and pressure are now achievable in the ultra super-critical range of 650o C and 375 bars. Two stages of steam reheat is commonplace.
For application in Bangladesh, we chose a modest range for unit size and thermo-physical parameters because of their high reliability and extensive experience throughout the world. The modest thermo-physical parameters we chose are 538°C and 165 bar steam temperature and pressure, respectively, and single steam reheat. We chose two common and standard unit sizes: 300 and 500 MW. We developed estimates of cost and performance for steam turbine based power plants using coal, and separately for those using natural gas.
5.1.1.2 Simple Cycle Combustion (Gas) Turbine (SCGT)
Gas turbine technology has been used by the aerospace industry for decades to power aircraft. Use of gas turbine technology for generation of power is relatively new compared to steam turbine technology. In a simple cycle configuration, where the exhaust gas from the turbine is released to the atmosphere without utilizing much of its energy, the technology is less efficient than steam turbine technology. The technology is best suited for burning natural gas.
Gas turbine technology has significantly improved over the past decade with respect to reliability, availability, and performance. The size of a single gas turbine unit has progressed to more than 300 MW. Similarly, simple cycle efficiency has improved and can now exceed 30%. The high capacity and efficiency has been possible due to the introduction of external features, such as inlet air cooling, inter-stage compressed air cooling, and recuperation.
Recuperation is the process of utilizing energy of the turbine exhaust gas to pre-heat the combustion air. High combustion temperature has also contributed to high efficiency.
For application in Bangladesh, we chose a modest range for unit size and external features because of their high reliability and extensive experience throughout the world. The two common and standard unit sizes we chose are 100 and 150 MW.
5.1.1.3 Combined Cycle Gas Turbine
Gas turbines are also used in a combined cycle configuration, where the exhaust gas from the turbine is used to generate steam, which in turn is used in a steam turbine to generate additional power. Thus, by burning the same amount of fuel, a combined cycle gas turbine (CC) system generates about 50% more power than a simple cycle gas turbine system. As a result, the efficiency of a combined cycle plant is approximately 50% higher than that of a simple cycle plant.
As a result of recent improvements in technology, the capacity and efficiency of the CC have greatly improved. The highest capacity combined cycle plant approached 1,000 MW. Combined cycle systems come in a number of configurations including 1x1, 2x1, 3x1, and 3x2. The first number in the configuration designation indicates the number of gas turbines and the second number indicates the number of steam turbines. Capacity split between the gas and steam turbines is nominally two-thirds and one-third of the total capacity, respectively.
The combined cycle gas turbine system has become the technology of choice for base-load generation wherever gas is available throughout the world. High fuel efficiency and relatively low capital costs make the technology attractive. Fuel consumption per megawatt- hour is the lowest of all generating technologies. Another attractive feature of the technology is that a CC plant can be installed in less time than typical steam turbine plants.
As the high efficiency and capacity achieved by these relatively new systems has not had long-term experience worldwide, for application in Bangladesh we chose a modest range of systems having an established technology base with extensive experience.
5.1.1.4 Nuclear
Nuclear plants offer the possibility of using a fuel source other than fossil fuels. Some countries without substantial fossil fuel resources have turned to nuclear power, especially France and Japan.
However, their capital costs are inherently higher than those for fossil plants. Their low steam conditions require larger equipment. They have systems not necessary in fossil plants, such as spent fuel handling and storage, radioactive gas handling and disposal, and others.
Concerns about safety extend the plant design process and add to plant costs. Concerns about security have a similar effect. In some countries public opposition has made it nearly impossible to site a nuclear plant, and in any event extends length and cost of the process.
Nuclear plant designs vary according to the coolant, which can be gas, light water, or heavy water; the moderator, which can be graphite, light water, or heavy water; and the state of the water, which can be boiling or pressurized and not boiling. Pressurized light water reactors
have gained a dominant position in the industry. For application in Bangladesh we chose a 500 MW pressurized light water reactor.
5.1.1.5 Generation Based on Renewable Resources
Generation based on renewable resources offers many benefits compared to fossil fuel or other conventional generation.
- Reduced reliance on fossil fuels that often are imported
- Reduced air emissions and other negative environmental impacts
- Protection against the exhaustion of non-renewable resources
- Provision of electricity in remote locations far from the main grid.
Hydro, wind, wood, sunlight, and agricultural waste have provided heat and power for centuries. More recently other resources such as electrical generation from solar, landfill gas, and municipal solid waste have been used commercially. Thousands of MW of capacity from all of these resources has been installed in the United States in the last twenty years and elsewhere. The challenges facing these resources include:
- High capital costs due to the diffuse nature of the resource (solar power)
- Lack of the basic resource (wind)
- Low fossil fuel prices
- Because of the above, much of the recent development outside Bangladesh was based on tax credits and other incentives which no longer exist
Fossil fuel prices have risen significantly in the last two years. This may eventually provide incentive for some renewable resource development in Bangladesh. However, over the last decade the availability of domestic natural gas and other factors have limited progress in the development of renewable resources for power generation. Some small projects have moved forward:
- BPDB has lately initiated a 1.8 MW wind energy project, presently under implementation near Feni
- REB as a part of its mandate to extend electricity to rural areas installed solar photovoltaic (SPV) power systems from 1993 to 1997
- BPDB implemented solar power applications including vaccine refrigeration, street lighting, agricultural pumps, mini grids, and railway signaling in three areas of Chittagong Hill Tracts
- Other initiatives include a five KW SPV power system for a fisherman’s village in Chokoria near Cox’s Bazar and four solar submersible pumping systems at Barendra that should be replicable in many other locations of the country
- Private enterprises and non-governmental organizations (NGOs) have installed a total of 58,500 home solar systems
- Another initiative involving a GOB owned financial institution has helped bring light to over 25,000 homes
- A 20 KW mini hydro generating unit at Bamachora implemented shows the promise of harnessing the hydro energy elsewhere in Chittagong Hill Tracts
Although these successes are encouraging, they are peripheral to the issue of generating bulk electricity for the main grid. We have not found any renewable resource generating options likely to be economic for that purpose.
It is worthwhile to note that the most successful application of energy efficiency if not renewable resources in the United States was the increased use of cogeneration. Substantial industrial demand for heat and the widespread availability of natural gas created an ideal environment for cogeneration. Adding gas turbines for power generation and waste heat boilers to capture the energy in the exhaust gas was economically feasible without tax or other incentives. This is not strictly within the control of the electric utility, and industrial demand may be limited. Nevertheless this technology may be the most promising for substantial power generation in Bangladesh.
5.1.2 Characteristics of Selected Generation Technology Options
Based on Nexant’s internal data base, review of recent literature, and extensive discussions with BPDB, we determined the characteristics of the generation technology options. Table 5- 1 presents the performance and cost parameters of the selected options.
Table 5-1 Generation Expansion Options
| | | | | | | | | Net Heat Rates at Grid, KCAL/KWH | | O&M Cost |
Unit Type | Net Unit Capacity (At Grid) | Thermal Parameters | Equipment Cost $/kW | Installation Cost $/kW | Total Project Cost $/kW | Construc-tion Time Per Unit, Months | Plant Life, Years | Minimum Load, % of Capacity | At 100% Power | At 75% Power | At 50% Power | Weeks/year Sched Maint | FOR, % | Fixed $/kW-month | Vari-able $/MWH |
Steam (coal) with FGD | 300 | Note 1 | 1,097 | 269 | 1,366 | 60 | 30 | 50% | 2,173 | 2,217 | 2,287 | 8 | 8% | 0.58 | 1.80 |
Steam (coal) with FGD | 500 | Note 1 | 896 | 215 | 1,111 | 60 | 30 | 50% | 2,154 | 2,198 | 2,268 | 8 | 8% | 0.58 | 1.80 |
CC (natural gas) | 300 | Dual Pressure | 438 | 232 | 670 | 36 | 25 | 50% | 1,720 | 1,856 | 2,023 | 6 | 6% | 0.42 | 2.00 |
CC (natural gas) | 450 | Triple Pressure | 361 | 232 | 593 | 36 | 25 | 50% | 1,686 | 1,819 | 1,984 | 6 | 6% | 0.38 | 1.80 |
CC (natural gas) | 700 | Triple Pressure | 322 | 180 | 502 | 36 | 25 | 50% | 1,564 | 1,688 | 1,840 | 6 | 6% | 0.38 | 1.80 |
SCGT (natural gas) | 100 | | 238 | 163 | 401 | 24 | 20 | 50% | 2,687 | 2,986 | 3,161 | 4 | 4% | 0.42 | 2.50 |
SCGT (natural gas) | 150 | | 227 | 122 | 349 | 24 | 20 | 50% | 2,605 | 2,894 | 3,064 | 4 | 4% | 0.42 | 2.50 |
Steam (natural gas) | 300 | Note 1 | 711 | 263 | 974 | 60 | 30 | 50% | 2,127 | 2,171 | 2,239 | 6 | 6% | 0.58 | 1.60 |
Steam (natural gas) | 500 | Note 1 | 579 | 211 | 789 | 60 | 30 | 50% | 2,109 | 2,152 | 2,220 | 6 | 6% | 0.58 | 1.60 |
Nuclear (light water reactor) | 500 | Note 2 | 1,739 | 978 | 2,717 | 60 | 40 | 50% | 2,598 | 2,651 | 2,735 | 6 | 8% | 1.67 | 0.50 |
Diesel (diesel fuel) | 10 | Medium Speed | | | 450 | 24 | 15 | 50% | 2,900 | 3,050 | 3,200 | 3 | 15% | 0.83 | 3.00 |
| | | | | | | | | | | | | | | |
Note 1: Superheat, Single Reheat, 165 bar/538 deg C/538 deg C | | | | | | | | | |
Note 2: Saturated, Steam Reheat, 73 bar/293 deg C | | | | | | | | | | | |
| | | | | | | | | | | |
5.2 TRANSMISSION OPTIONS
Whereas generation options define sources of energy, and hence focus on fuel economics, availability, and energy conversion efficiency, transmission options define means of transferring generated energy to where it is consumed. Transmission options are location and path dependent, much more so than generation options. For transmission options, the focus is on amount of power to transfer, distance to transfer, and the continuing reliability of the interconnected system.
Since the function of transmission is to connect generation to load, the options for transmission development must address two significant uncertainties. First, there is the uncertainty of where and how fast energy demand grows. Second, there is the uncertainty of where the generation will be constructed, when, and what size. These types of uncertainties offer a broad variety of possible combinations resulting in numerous future scenarios.
However, neither are unbounded uncertainties; there are physical limits to how much load and generation can be added or located in specific areas of the power system. Furthermore, the uncertainties, in as far as they would influence transmission options, are further bounded by the consequence of location. For example, if generation is located close to load, then the transmission requirement is less than if generation is located far from load. To extend this concept further, if generation is located where load was previously supplied via the transmission system, then the use of existing transmission is reduced, further extending the time when such transmission will need reinforcement. Transmission options can thus be defined in terms of minimum and maximum transmission requirement, relative measures of the amount and type of transmission expansion needed to accommodate future growth of the power system.
In the specific case of the Master Plan study, the generation expansion plan and the load growth forecast define a future scenario that in turn defines the transmission options. In order to address the maximum (bounded) uncertainty, we first focus on the far future condition, the so-called horizon year. In broad strokes, it is possible to assess what transmission options should be considered by conducting network analyses of horizon year conditions. For instance, by identifying major pockets of load and how demand growth will be supplied to each load pocket would determine the voltage level for future transmission expansion.
As an example, say that the Dhaka load grows by 10,000 MW from 2005 to 2025, and new generation will supply 8,000 MW of this growth in the Dhaka region. The additional transmission capacity into Dhaka is then 2,000 MW. The typical capacity for transmission lines by voltage level is:
- 750 KV: 5,500 MVA/circuit
- 400 KV: 2,500 MVA/circuit (for 4 conductors per circuit)
- 230 KV: 600 MVA/circuit (for 2 conductors per circuit)
- 132 KV: 140 MVA/circuit (for 2 conductors per circuit)
Based on the above, 750 KV development is far beyond the needed capacity, while 132 KV will require many circuits to support the future growth of Dhaka. Hence the transmission options are 230 KV and 400 KV for future supply of the Dhaka region.
A similar process is applied in other load centers of PGCB to identify transmission options to support regional development.
Another consideration in determining transmission expansion options is the ability of certain options to address broader issues. This is another reason for first developing a transmission system for a horizon year like 2025, then developing the system for each earlier year with full knowledge of what the system might look like in 2025. The advantage of this approach compared to developing the transmission system in chronological order (first for 2010, then for 2015, etc.) is that one avoids installing components that later become redundant, or worse, committing to a course that is well below optimal for the long term.
Another type of broad issue is the placement of new generation given that the location of new generation is within the utility’s control. Two natural possibilities for Bangladesh are to site new generation at load centers, to minimize electric transmission costs, or to site new generation near gas fields, to minimize gas transmission costs. Ideally one would seek a least cost solution considering both electric and gas transmission costs. However, determining a joint least cost gas and electric transmission development scheme is beyond the scope of this study. Instead, the process we have applied is to develop a generation plan out of a set of viable options, and from the resulting plan, develop a transmission plan.
As noted earlier, higher voltages can handle large power flows. In addition, higher voltage lines have lower unit cost, less losses, and less right-of-way requirements. However, Bangladesh is not large geographically, is not interconnected with other countries, has and will have substantial generation located close to the Dhaka load center, and thus will not be moving large blocks of power around the country. These offsetting factors make the analysis of a move to 400 KV a worthy topic for this study.
Accordingly, this study’s analysis of transmission options included the following steps.
- Evaluating 230 KV development vs. development that would use more 400 KV components, in addition to the already PGCB committed 400 kV lines, where they would be expected to be most cost-effective.
- Determining the unit cost of transmission system components for use in the above-mentioned evaluation of two options, and for estimating the cost of the developed systems in 2010, 2015, 2020, and 2025 for the financial and economic analysis discussed in Section 8.
Further aspects of uncertainty are addressed by conducting sensitivity analysis after a transmission plan has been determined. For example, the alternate location of generation, or alternate dispatch, which may impact transmission requirements, are addressed by developing additional simulation models of these conditions, and analyzing them assuming that a base transmission plan is in place. Any additions to the base transmission plan are then identified from the analysis.
A broader assessment of alternative generation development was not conducted since such was beyond the scope of the present work. However, such alternatives may also be addressed via sensitivity analyses, or by scenario planning techniques.
5.2.1 Transmission Expansion Options
For the Master Plan study, the expansion options comprise:
- 400 KV AC lines: 4x795 MCM ACSR conductors per phase
- 230 KV AC lines: 2x795 MCM AAAC conductors per phase
- 132 KV AC lines: 636 MCM AAAC conductor per phase
These voltage levels were identified as a result of the conducting broad stroke analysis of the horizon year conditions following the generation expansion plan and load forecast for the Master Plan. Hence, the transmission options were identified after the generation expansion planning and load forecasting efforts of the work.
For congested urban areas, such as Dhaka, where new right-of-way might be difficult or impossible to obtain, we considered voltage uprating as a development option. In this option, the existing 132 KV line is retained, with modifications to allow for operation at 230 KV. In practice, the feasibility of the uprate will depend on specific characteristics of each uprated line. For planning purposes, we assume that the uprate is feasible. If it does bear out to be infeasible, the alternative is to replace the existing 132 KV line with a new 230 KV line on the same right-of-way. There is an additional cost impact to this that would need to be considered at time of implementation.
The options above did not include others that might be considered in a similar Master Plan study for specific reasons.
- High-voltage DC lines. The terminal costs for these lines tend to be relatively high, and thus these lines are economically viable where the application is for distances of 200 KM or more for transfers of 1,000 MW or more.
- Other voltage levels such 500 or 345 KV. The typical approach is to double the voltage level to take advantage of economies of scale. However, at 500 KV, we would have more capacity than needed for the horizon year, and at 345 KV, not enough capacity. The 400 KV level was reasonable choice for next voltage level to include amongst the options.
For transformers, we aimed to standardize future additions to the following sizes:
- For 230/132 KV transformers: 225/225 MVA normal/post-contingency rating for units consisting of 3 single-phase units
- For 400/230 KV transformers: 375/375 MVA normal/post-contingency rating consisting of 3 single-phase units
Where existing transformers of different capacity were at the substations, we considered as an option adding future transformers of the same capacity as the existing units. This is to avoid loading imbalance and circulating currents on the transformers when they operate in parallel.
5.2.2 Costs of Transmission System Components
For assessing the cost and economic impact of transmission options, we used cost data for 2005 as provided by PGCB. Where such data was not available, we used typical costs from similar options applied in other countries worldwide.
For transmission lines, we used the following 2005 costs:
- 400 KV, double circuit: BDT 20.0 million per KM
- 230 KV double circuit: BDT 13.0 million per KM
- 132 KV double circuit: BDT 7.0 million per KM
For single circuit lines, we assumed 65% of the cost of a double-circuit line of the same voltage level. Also, where a double-circuit line is proposed, but stringing only one circuit initially, we assumed that the cost of stringing only one circuit of a double-circuit line is 65% of the cost of a double-circuit line of the same voltage level. The subsequent stringing of the second-circuit is assumed to cost 35% of the cost of a double-circuit line at the same voltage level.
For transformers, we used the following 2005 costs:
• 400/230 KV, 375/375 MVA: BDT 287.0 million
• 230/132 KV, 225/225 MVA: BDT 127.6 million
• 230/132 KV, 125/125 MVA: BDT 63.8 million
Each new transformer and transmission line added to the system requires corresponding terminal equipment. The most expensive component of termination equipment is circuit breakers. We assumed the following costs for circuit breakers, including installation and site preparation work:
- 400 KV circuit breakers: BDT 79.8 million
- 230 KV circuit breakers: BDT 54.2 million
- 132 KV circuit breakers: BDT 22.3 million
Substation configurations were typically breaker and a half, with a few exceptions. This configuration would require 3 sets of circuit breakers for every 2 terminations.
Manually switched capacitors were assumed to cost BDT 0.45 million per KVAR. These are applied in the plan to provide control for steady-state voltages.
As the demand grows at existing 132/33 kV substations, they will become overloaded and new substations will be required. These substations and connecting lines are a substantial part of the overall cost of the transmission system that we wanted to incorporate in our cost analysis. Locating these new 132/33 kV substations and lines was beyond the scope of this project. However, we could estimate the number needed based on the demand and the capacity of existing substations. We emphasize that our results were not the product of load flow or other technical analysis. We simply compared the demand at each substation to its capacity, and added a new substation when the demand exceeded the capacity.
We developed estimates of the associated costs of the substations and lines. Based on data from PGCB we used the estimates below for the cost of each substation and connecting 132 kV transmission lines.
Each “standard” substation would include two 50/75 MVA transformers, four 132 kV line bays, two transformer bays, a breaker and a half scheme, two 33 kV bays, and land. The associated transmission line requirements were assumed to be 30 km of 132 kV double circuit lines, with 636 MCM ACSR conductor and OPGN. The exchange rate used was 67 Taka/US$.
- Substation cost: BDT 283.56 million
- Line cost: BDT 173.20 million
- Total cost: BDT 543.36 million
5.3 GENERATION SITING OPTIONS
5.3.1 Introduction
BPDB’s system is almost entirely thermal based and is expected to be so in future. The only hydro plant is located at Kaptai, in the south-eastern region of the country with an installed capacity of 230 MW and there is no viable hydro potential at any other site. The potential sites for installation of new generating plants during the study period of the PSMP (2005- 2025), were assessed considering the vacant space available at the existing sites; the scheduled retirement dates of the existing units, and the replacement of these units by planned units of appropriate size; and new sites based on the normal sitting criteria. With regard to thermal plant sites, various locations in all the regions of both the East Zone and West Zone were included in the evaluations, as well as alternative sources and types of fuel, and appropriate generation technologies.
5.3.2 Siting Criteria
The thermal power plant sites are selected based on a number of factors. Such factors include the following.
- Proximity to the load centers and their forecast load demand.
- Transmission to the load centers.
- Availability of adequate space at the site.
- The value of the land for other uses.
- The suitability of the ground and geotechnical conditions for construction of the plant.
- The possibility of flooding or seismic events.
- Potential sources of cooling water and makeup water.
- Fuel deliverability at the site.
- The impact of the facility in a positive or negative manner on the local environment.
- Sources of fill and construction materials.
- Access to the site for transportation of heavy equipments and construction materials.
- Availability of social facilities near site.
Site rankings are established based on consideration of the above factors. The rating process consists of qualitative judgments on factors that cannot be measured in a quantitative manner.
5.3.3 Existing Sites
The existing power plants are concentrated in the East Zone of the country because of the availability of indigenous natural gas in this Zone. The gas is supplied to the power plants through the National Gas Transmission Network. The larger plants are installed near Dhaka, the largest load center of the country, accounting for about 50% of total demand of electricity. The existing and committed power plant sites are described below. The sites, where space is available for further addition of generating units due to availability of vacant space or retirement of existing units during the planning period are also indicated. All the sites are owned by Bangladesh Power Development Board (BPDB) except as mentioned otherwise.
5.3.3.1 Dhaka Region:
Ashuganj: The existing site is located 60 KM northeast of Dhaka on the bank of Meghna river, one of the largest in Bangladesh. There are 2x64 MW steam turbine plant, 3x150 MW steam turbines plant, 1x90 MW CC and 1x64 MW SCGT. All the units are gas based. The 2x64 MW steam units are scheduled to be retired in 2008. The 90 MW CC and the 60 MW SCGT plants are scheduled to be retired in 2010. The 3x150 MW ST units will be retired by 2023. 1x700 MW CC plant and 1x150 MW SCGT plant may be installed in the vacant spaces of the retired units.
Ghorasal: The existing site is located 25 KM north east of Dhaka on the bank of Sitalakhaya river. The two 2x55 MW steam turbine plants were installed in 1974 and 1976 and these are scheduled to be retired by 2012. In this space, a new 700 MW CC plant may be installed. The 4x210 MW steam turbine plants were installed during 1986-1998 and none of these units will retire during this planning period. All the units are gas based. There is no space for further expansion.
Haripur: The existing site is located about 15 KM southeast of Dhaka on the bank of Sitalakhaya river. There are 3x33 MW gas based SCGTs installed in 1987. These are scheduled to be retired in 2010 and 1x150 MW SCGT may be installed in this space. There is a plan to install a gas based 150 MW CC plant at the existing site which is expected to be commissioned in 2010. There is no space for further expansion.
Meghnaghat: This is a large site 22 KM southeast of Dhaka on the bank of Meghna river. The site has been developed by BPDB to accommodate at least 4 large gas based power plants. Presently a 450 MW gas based CC plant has been installed in 2003 by IPP. A second 450 MW gas based CC plant, to be developed by IPP is committed. Thus, there is space for addition of two more gas based CC units of total capacity of 900 MW.
Siddhirganj: This large site (1.5 KM x .5 KM) is only about 11 KM southeast of Dhaka, on the Sitalakhaya river. A 210 MW gas based steam turbine plant has been installed recently which will not be retired within the planning period. 3x120 MW gas based SCGTs are committed to be installed there. Another 210 MW ST unit is planned to be installed. There should be adequate space for further addition of a 450 MW CC plant.
Konabari (North Dhaka): The Rural Power Company (RPC) has planned to install a 450 MW gas based CC plant at this location. The plant is expected to be commissioned by 2009.
5.3.3.2 Central Region
Fenchuganj: The site is located in the north-eastern part of Bangladesh on the river Kushiara. There is a gas based combined cycle plant of 90 MW (2x30 MW SCGT + 1x30 MW ST) capacity which is scheduled to be retired by 2022. Two 150 MW SCGT plant may be installed in the same space. Another 90 MW CC is committed to be installed by 2008.
There is no further space for addition of any other unit. The river water is not adequate, particularly during dry season for cooling of the plant and hence a cooling tower has been installed.
Shahjibazar: There are 7 numbers of small gas based SCGTs (commissioned in 1969) with a total present capacity of 96 MW. These units are scheduled to be retired by 2006. Another 2x35 MW SCGT plant was installed in 2001 which will retire by 2023. A number of SCGTs of total capacity of 150 MW may be installed in this space. There is no further space for installation of any other unit.
Sylhet: The existing 20 MW gas based SCGT is scheduled to be retired by 2008. A 100 MW SCGT is committed to be installed at the site by 2007.
Mymensing: The site is owned by Rural Power Company, an IPP. Gas based 4x35 MW SCGTs exist at the site. 2x35 MW ST will be installed soon. The plant is not scheduled to be retired within the planning period. There is no space for addition of any other unit.
5.3.3.3 Southern Region
Chandpur: BPDB has planned to install a gas based 150 MW CC plant. The site is about 120 KM south-east of Dhaka, near Meghna river. At present, 100 MW SCGT is committed to be installed by 2007.
Kaptai: This is the only hydro power plant in the country, located in the south-eastern region. The existing units are 2x40 MW and 3x50 MW. Another 2x50 MW units are planned to be installed at the site. There is no space for further expansion.
Rauzan: There are 2x210 MW gas based ST units (installed in 1993 and 1998) which will not retire within the planning period.
Sikalbaha: There is one 60 MW gas based ST unit and 2x28 MW gas based SCGT (barge mounted) units. The condition of the barge units is not satisfactory and are scheduled to be retired in 2006 and the ST unit will retire in 2013. A 450 MW CC unit and one 150 MW SCGT may be installed at the site after retirement of the old units. There is no further space at the site for addition of any new units.
5.3.3.4 Northern Region
Baghabari: The gas supply has been made available to this power station in 2002 after the national gas transmission network was extended to the West Zone through the pipeline on the Bridge over the Jamuna river. 1x71 MW SCGT and 1x100 MW SCGT are installed at the site. The 71 MW unit will be retired in 2013. The 100 MW SCGT will retire in 2022. 2x150
MW SCGT may be installed in this space. There is no further space for expansion. There is also a Barge Mounted IPP plant of 2x45 MW (SCGT) capacity. A 40 MW ST will be added to these SCGTs to make it a combined cycle plant of 130 MW capacity. Another 130 MW barge mounted CC plant will be installed by the same IPP by 2006.
Sirajganj: This site is on the bank of river Jamuna, about 120 KM north-west of Dhaka. The site, now under development, is owned by BPDB. Installation of a 450 MW CC power plant by IPP is in the bidding process. The plant is expected to be commissioned by 2008. The fuel will be gas which will be supplied through the nearby gas transmission network. There will be adequate space for installation of another 450 MW plant. The Power Grid Company of Bangladesh (PGCB) is constructing a 230/132 KV grid substation adjacent to the site.
Bogra: BPDB has planned to install a 450 MW CC plant around Bogra. Gas transmission network will be extended to the area by 2006. However, a new site is required to be acquired and developed.
Barapukuria: The site is near the Barapukuria coal mine in the northern region of Bangladesh. BPDB is installing 2x125 MW coal based power plant at the site. The 1st unit of the plant is expected to be commissioned in November, 2005 and the 2nd unit in March, 2006. There is space for addition of 1x125 MW unit at the site.
Rangpur: There is 1x20 MW oil (diesel) based SCGT at the site which will be retired by 2009 as per plan. A 150 MW SCGT may be installed at the site. Addition of further units are not considered presently as there no gas supply yet in the area and there are also limitations in transportation facilities for larger units.
Saidpur: There is 1x20 MW oil (diesel) based SCGT at the site which will be retired by 2008 as per plan. A 150 MW SCGT may be installed at the site. Addition of further units are not considered presently as there no gas supply yet in the area and there are also limitations in transportation facilities for larger units.
5.3.3.5 Western Region
Khulna: The site is in the 3rd largest load center of the country and located on the Bhairab river. There is no gas supply in the area yet. However, there is plan to extend the national gas transmission network to Khulna by 2010.There are 1x60 MW and 1x110 MW oil (HSFO) based ST units, and also 2x28 MW barge mounted SCGT units at the site. There is also a barge mounted IPP power plant of 110 MW capacity at the site. BPDB has also planned to install a 210 MW oil fired ST power plant at the site for which adequate space is available.
The existing 60 and 110 MW units are scheduled to be retired by 2009 and 2019 respectively. In this space, a 450 MW CC plant may be installed after gas is available at the site. There is no space at the site for further expansion.
Barisal: There are 2x20 MW Diesel fired SCGTs which will be retired by 2009. 1x150 MW SCGT may be installed at the site after retirement of the existing units. However, it is not certain when gas network will be extended to Barisal.
Bhola: BPDB has planned to install a gas based 150 MW CC plant at this location. A gas field exists near the site.
Bheramara: There are 3x20 MW Diesel fired SCGTs which will retire by 2008. It is planned to extend the national gas network to Bheramara by 2010. Therefore, gas based 1x150 MW SCGT may be installed in the space of existing units. BPDB has planned to install a gas based 450 MW CC plant near the existing site.
5.3.4 Potential New Sites
As described above, the existing sites have limited space for further expansion. Therefore, it would be necessary to develop new sites to accommodate a large number of new plants to be installed within the planning period (2025). The PSMP 95 has been reviewed in this respect. Based on the regional demand, availability of fuel, availability of cooling water, transportation facilities and other power plant siting criteria and the review of PSMP 95, a number of potential sites have been identified in the different regions of the country as described below. However, it is to be noted that detailed feasibility studies would be necessary to confirm the suitability of the sites.
5.3.4.1 Dhaka region
New Meghnaghat/Daudkandi: A new site may be developed for 2000 MW CC plant, at a suitable location in the area. The advantages are: (1) the location is near to the largest load center, Dhaka (about 22 KM from Dhaka) as well as Comilla, a large load center, (2) the location is near the major gas supply system and 230 KV grid network, (3) it is on the bank of a large river, Meghna which will provide adequate cooling water as well as facilitate transportation of heavy equipment.
Aminbazar/Dhaka West: The location is on the western periphery of Dhaka city. A new site may be developed for installation of 2x450 MW CC and 2x150 MW SCGTs. The site has a special advantage of being located near the western part of the Dhaka 230 KV ring, which will enhance the reliability of power supply and also improve the voltage profile. It may be noted that all the power plants near Dhaka are located on the north-east and south-east of Dhaka.
Mawa: The location is about 50 KM south-west of Dhaka. A suitable site (about 100 acre) may be acquired and developed near the proposed Mawa Bridge over the large river Padma. There is provision for laying high voltage electric cable and gas pipeline over the bridge. The electric cable over the bridge would greatly facilitate establishing the third electrical inter- connector between the East Zone and the West Zone. Therefore, a large power plant of 2800 MW capacity at this location would be very useful. Further, this power plant would supply power to Dhaka from the western side of the city and will greatly enhance the reliability of the power supply as the power plants supplying Dhaka city are concentrated on the eastern side of Dhaka.
5.3.4.2 Central Region
Mymensingh: A new site may be developed to install 3x150 MW SCGTs. Gas supply is available at the location.
Sylhet New: A new site may be developed to install 2x150 MW SCGTs.
5.3.4.3 Southern Region
Chittagong New: Chittagong is the second largest load center in the country. Therefore, new sites will be required to be developed to install power plants required during the planning period. Probable sites could be near Madanhat or Sikalbaha area where at least 2000 MW CC and 500 MW SCGTs may be installed.
Chittagong New: (Coal): In case sufficient gas is not available, imported coal based power plants may be required to be installed at this location. A suitable site will be required to be acquired to accommodate 3500 MW power plant.
Feni: A new site may be developed to install a 150 MW SCGT.
5.3.4.4 Northern Region
Rajshahi: A new site on the bank of the Padma river may be developed for installation of about 450 MW gas based CC power plant. The gas transmission network is planned to be extended to the area by 2010.
Barapukuria/Phulbari: There is possibility of developing coal mine at Phulbari, near Barapukuria, which may provide fuel for about 2500 MW coal based power plant. A suitable site may be acquired and developed near the coal mine.
5.3.4.5 Western Region
Khulna: A new site on the bank of Rupsa river may be developed for installation of about 2000 MW gas based CC plant. It is expected that gas supply would be available by 2010.
Mongla: In case imported coal/oil option is required to be adopted for power generation, this would be a very suitable location, as it is the second sea port of the country. A suitable site may be developed to install about 5000 MW coal based power plant.
5.3.5 Summary
Table 5-2 summarizes the sites selected for generation capacity additions at existing, planned, and potential new sites in different regions during the planning period, for the Base Case scenario.
Table 5-2 Summary of Sites and Capacity Additions for Base Case
Region | New Capacity Additions (MW) |
Existing Sites | Planned Sites | New Sites | Total |
Dhaka | 2,992 | 450 | 6,500 | 9,942 |
Central | 407 | 0 | 900 | 1,307 |
Southern | 799 | 150 | 2,600 | 3,549 |
Northern | 1,450 | 450 | 600 | 2,500 |
Western | 897 | 450 | 1,850 | 3,197 |
Total | 6,545 | 1,500 | 12,450 | 20,495 |
Section 6 Generation Expansion Plan
6.1 INTRODUCTION
6.1.1 Objectives
The objective of Component B is to develop an updated version of the generation and transmission expansion plans for Bangladesh. Our general approach is to assist BPDB, PGCB, PMU, and other Bangladeshi organizations in conducting the work, and collaborate with them in preparing this report. Several steps reported on in previous sections are involved in preparing the generation expansion plan, including:
- Develop data base on the existing system (Section 2).
- Prepare load forecast (Section 3).
- Evaluate the price and availability of power plant fuels (Section 4).
- Establish generation expansion options and sites for new generating units (Section 5).
This Section 6 presents the generation expansion plan. Our overall objective for this Task is to develop a least-cost generation expansion plan for the Bangladeshi power system covering the period 2005-2025. The generation expansion plan identifies the size, technology, fuel, and timing for new generating plants. The process of establishing the plan also permits development of large data sets tabulating the costs, fuel use, reliability, and other factors useful in analyzing issues relevant to decision-making.
Several sub-objectives support that overall objective.
- Establish a base case scenario and corresponding generation expansion plan for mid-range or most likely conditions. This provides a reference point for further analysis.
- Evaluate the impact of changes to base case conditions through development of a set of scenarios. If the resource plans for different cases are similar over a range of conditions in input variables such as demand or fuel price, one can have more confidence in starting with implementation of the base case plan. Changing conditions are less likely to mandate changes to the resource plan that would be too disruptive or costly. Furthermore, evaluating scenarios can also demonstrate the costs or benefits and other consequences of making choices in areas where choices are possible, such as fuel type.
- Determine the value of the energy and capacity supplied by generation options to establish an upper limit on what should be paid to construct and operate them. Where the cost, in particular the construction or fuel cost, of a generation option is especially uncertain, it can be extremely useful to determine the value of the energy and capacity provided by that option. This is accomplished by calculating the cost of replacing those services, which often can be done easily with the analytical tools used for generation planning. This replacement cost is an upper limit on what should be paid to construct and operate the option.
6.1.2 Approach
In developing optimal generation expansion plans, we used two complementary kinds of analysis. The starting point for both of them is comprehensive data about Bangladesh’s power system, including the costs and performance of existing and potential new generating units.
The first part of the analysis used screening curves. The method consists of developing generation cost curves that show the type of unit that is most economical at each capacity factor. Screening curves plot the annual total cost of electricity from a unit over the range of capacity factors from 0% to 100%. Capacity factor is defined as actual output divided by maximum possible output. For example, a unit with a rating of 100 MW that generated 613,200 MWH in a year would have a capacity factor of 613,200/(100 * 8,760) = 70% for that year. One type might be most economical at low capacity factors and would be most suitable for peaking duty. Another might be most economical at high capacity factors and would be most suitable for base load duty. The curves provide a convenient initial comparison of different technology and fuel types.
System operators can dispatch fuel-burning units so that, for example, one using high price fuel would achieve a low capacity factor and another with low price fuel would achieve a high capacity factor. Hydro is different in that the output is limited by the available water. The operators have little influence over a hydro unit’s capacity factor, so a hydro plant typically appears as a single point on the graph, corresponding to the capacity factor associated with an average water year, rather than as a line.
The second part of the analysis used a sophisticated computer program for power system optimization and simulation to develop optimal generation expansion plans for the Bangladeshi power system. Based on extensive inputs about the existing system, future conditions, and characteristics of candidate technologies for generation expansion (new power plants), the optimization program provides answers on the type, size, and timing of plant additions during the planning period. The goal is to install new generation capacity on an economic basis while maintaining system reliability. The difference from the screening curve analysis is that in this analysis the technology type and capacity decisions also depend on the size of the units relative to the existing power system, how the new units are to be operated among the existing generating units, the optimal level of reliability, and on the future load growth.
6.2 POWER SYSTEM RELIABILITY AND ENERGY NOT SERVED (ENS)
6.2.1 General
In Bangladesh today, the power system is unreliable. Often, not enough generation is available to serve demand. BPDB sheds load by disconnecting distribution circuits on a rotating basis throughout the country. Thus, many customers occasionally have electricity available for fewer than 24 hours per day. It is clear that more generation must be built to supply customer demand.
A key question is how much generation is enough to supply customer demand with appropriate reliability. It could be argued that 1,000 MW of installed generation is needed to meet a peak demand of 1,000 MW, but this ignores the need for operating reserves and the chance that some of the 1,000 MW of generation would not be available due to scheduled
(planned) or unscheduled (forced) maintenance outages. These outages keep any individual unit out of service from 10-25%, or more, of the time. Thus, the power system needs substantially more than 1,000 MW to meet demand of 1,000 MW. Units experience random forced outages at rates of 10-15% of the time in our analysis. Typically, power system operators do not schedule outages during times when peak demand is likely to occur. Thus, the forced outages become the dominant factor.
Suppose that the power system installed twenty 100 MW units (total of 2,000 MW) with 15% forced outage rates to meet a peak demand of 1,000 MW. We assume forced outages to be random, so on average about 2,000 * 15% = 300 MW of units will be forced out of service.
However, there is some very small chance that more than 1,000 MW of units will be out of service at the time of peak demand, leaving the system unable to meet all demand. No matter how many units are installed, there will always be some chance that enough units will be forced out of service that the system is unable to meet all demand. As more units are added, the chance of this occurring becomes very small, but they are never zero.
Reserve margin is a measure of the generating capacity available over and above the amount required to meet the system demand requirements. It is defined as the difference between the total installed generating system capacity and the annual peak demand, divided by the peak system demand. For example, if total installed capacity is 1,200 MW and the peak demand is 1,000 MW, the reserve margin is 20%. This deterministic reliability index does not directly reflect system parameters, such as generation mix, unit size, and forced outage rate, but it does provide a reasonable relative estimate of reliability performance when parameters other than reserve margin remain constant. System planners and operators develop what they believe to be an adequate reserve margin for their system by monitoring system performance over time. Before probabilistic reliability measures were developed, reserve margin was the primary reliability index used by system planners.
Loss of load probability (LOLP) is a reliability index that indicates the probability that some portion of the load will not be satisfied by the available generating units. More specifically, it is defined as the proportion of days per year or hours per year when insufficient generating capacity is available to serve the load. Generation expansion planning programs such as WASP use complex procedures to calculate LOLP.
The LOLP is usually expressed as a ratio of times; for example, 0.1 days per year equals a probability of 0.000274 (i.e., 0.1/365). The target LOLP frequently used in the US for large interconnected systems is one day in 10 years. It depends primarily on the amount of installed capacity, unit sizes, forced outage rates, and level of demand. The target in Bangladesh used in the 1995 PSMP was 1.4% from 2005 to 2015, after sufficient time had passed from the 1995 start year to permit a relatively stable, reliable system to be built.
Earlier years were much less reliable, but trended down in LOLP from about 10% in 2000 to the 1.4% target by 2005. In our analysis we used a requirement of 1% from 2009 – 2025. In earlier years the LOLPs were much higher because the new plants being added were not sufficient to reach the out-year 1% requirement, and we believed there was not enough time to build more new plants.
The traditional reliability approach in optimization analysis is to use reliability criteria as constraints in the long-range generation expansion planning and to optimize an economic objective (e.g., minimizing total discounted system costs) subject to a specified reliability
criterion (e.g., reserve margin or LOLP). This approach to expansion planning does not, however, allow reliability-cost trade-offs. That is, it does not take into consideration the trade-offs between economic factors and different levels of reliability inherent in capacity expansion planning.
For our analysis we use a LOLP criterion, but add an approach that does permit direct trade- offs between the benefits of increased reliability and the costs of achieving that level of reliability. Among the key factors in this approach are the amount of ENS and the unit cost of ENS. The power system optimization and simulation program readily calculates the amount of ENS in a manner similar to the way LOLP is calculated, but it uses one that takes into account the probability of different amounts of shortfall each hour. The program adds new generating resources to lower the overall levelized system costs. At some optimal point, adding another generating unit adds more costs than the benefit of reduction in ENS and its costs, and the overall costs rise.
However, if the LOLP criterion is governing (i.e., requires higher levels of reliability than the strictly economic approach would), then the resource plan does not achieve the ideal least- cost balance of cost and reliability.
The overall cost of ENS is the amount of ENS times its unit cost. The unit cost is a measure of the economic impact of not meeting the electricity demanded. In other words, the unit cost of ENS is the costs that a potential consumer does incur when electricity is not available that he would avoid if electricity were available, divided by his amount of ENS. This economic impact comes from interruptions to industrial output, commercial activity, residential services, and public safety. Some interruptions are extremely costly, for example, when a batch of product is lost due to loss of power at an industrial plant. Others are more matters of inconvenience. Some are inherently difficult to quantify but possibly quite large, for example, the reduction in public safety due to loss of emergency services and increased frustrations with the lack of electricity. Surveys in industrial countries have produced values for the unit cost of ENS exceeding $10 per KWH of ENS. Keeping in mind these difficulties, two points regarding the unit cost of ENS are apparent:
- The unit cost of ENS must be higher than the price consumers pay for electricity. The value of the electricity to consumers is at least as high as the price they pay, because they are free not to use (and have to pay for) the electricity. This principle does not provide much guidance in Bangladesh, however, because prices to consumers are low and below the cost of production for new units. In industrial countries the prices are often above $0.10 per KWH.
- The unit cost of ENS must be higher than the cost that people incur to supply their own backup energy. Many facilities in Bangladesh rely on backup diesel generators to serve their needs when the power from the grid is not available. Based on the data in Tables 5-1 and diesel fuel, we calculated the cost of production from a 10 MW diesel unit. The analysis showed that cost of production is about $0.20 per KWH for operation 526 hours per year (6% capacity factor) and $1.00 for operation 70 hours per year (0.8% capacity factor). See Table 6-1.
Table 6-1 Cost of Production – Diesel Backup Generation
Capacity Factor, % | Hours/Yr Operation | Energy Production Cost, $/KWH |
0.0% | 0 | |
0.2% | 18 | 4.42 |
0.4% | 35 | 2.25 |
0.6% | 53 | 1.52 |
0.8% | 70 | 1.16 |
1.0% | 88 | 0.95 |
4.0% | 350 | 0.29 |
5.0% | 438 | 0.25 |
6.0% | 526 | 0.22 |
6.2% | 543 | 0.22 |
6.4% | 561 | 0.21 |
6.6% | 578 | 0.21 |
6.8% | 596 | 0.20 |
7.0% | 613 | 0.20 |
In the context of the overall electric system, the supply of reliable electric service to customers depends not only on the generating system but also on transmission and distribution systems. In fact, in industrial countries generation normally contributes little or nothing to overall ENS. Distribution outages are the primary cause of ENS, with transmission outages a secondary cause. All three of the subsystems (generation, transmission, and distribution) are important in considering how to reliably deliver electricity to customers. This report addresses generation planning and the reliability of the generating system. Planning and operating criteria, not economic tradeoffs, provide for the reliability of the transmission and distribution systems. Thus, the reliability of the three systems cannot easily be compared on a common basis.
6.3 SCENARIO DEVELOPMENT
6.3.1 Base Case Scenario
We developed the Base Case scenario and corresponding generation expansion plan based on mid-range or most likely conditions to provide a reference point for further analysis. The power system optimization and simulation program requires thousands of inputs. A few of those inputs or categories of inputs are more important than others in that their values in the future are uncertain and the differences in the values might have a significant impact on the results.
6.3.2 Scenarios to Evaluate the Impact of Changes to Base Case Conditions
We studied the scenarios discussed below using the WASP production simulation / system optimization program. We conducted screening analysis on additional scenarios discussed in Section 6.4
6.3.2.1 Demand
The demand forecast will affect the timing and amount of new generation needed and may affect the generation expansion plan’s optimal choice of technology and size of units. We
developed generation expansion plans for the three demand forecasts discussed in Section 3 and summarized below:
- Medium or Base Case: Demand growth averages 7.9% per year from 2025 – 2025, reaching 100,083 GWH and 19,312 MW in 2025 on a net generation basis.
- High Case: Demand growth averages 12.0% per year from 2025 – 2025, reaching 217,137 GWH and 41,899 MW in 2025 on a net generation basis.
- Low Case: Demand growth averages 6.7% per year from 2025 – 2025, reaching 80,982 GWH and 15,626 MW in 2025 on a net generation basis.
The level of future demand is not within Bangladeshi decision-makers control. An improved approach to pricing electricity and expanded demand-side management (DSM) efforts would influence but not control future demand. Thus, we analyze the impact of varying levels of demand to determine how the resource plan changes with changes in demand. If the resource plans for different demand levels are generally similar, one can have more confidence in starting with implementation of the base case plan and make adjustments in the future to accommodate the actual level of demand.
6.3.2.2 Availability of Natural Gas
Based on the cost of natural gas and coal plants and fuel, natural gas fueled plants are the clear economic choice. Our Base Case assumes that enough additional gas fields will be discovered and developed to supply gas to all Master Plan gas-fueled plant additions.
However, Bangladesh’s natural gas supply may be lower than we have assumed, or may be reserved for other purposes or extended through limited use. As alternatives, we consider three scenarios in which new coal units enter the resource mix.
In the first two we assume that natural gas is sufficient to fuel only all gas-fueled plants to be installed through 2016. In the first limited gas scenario, coal plants provide new generation starting in 2017. We assume domestic coal supplies sufficient to serve 4,000 MW of coal plants will be developed. Imported coal will supply plants after that limit is reached. We assume that gas will still be available for combustion turbines for peaking duty. In the second limited gas scenario, we add the same amount of coal-fired capacity, but spread the dates of operation over the period 2012 – 2025 rather than 2017 – 2025, which may be a more reasonable approach to the introduction of coal fueled units.
In the third scenario with coal units, we assume that Bangladesh chooses to install coal units even though natural gas is available. This would provide additional fuel security compared to an all natural gas approach. We install 4,000 MW of coal units over the period 2012 – 2023, all fueled with domestic coal.
The level of demand is largely outside the control of Bangladeshi decision-makers. We study variation in demand to evaluate its impact on the generation expansion plan, not to guide policy choices. In contrast, fuel type can be a policy choice affecting the Bangladeshi power sector, within the control of decision-makers. One choice is to exploit natural gas to the extent possible as quickly as feasible. Another is to diversify fuel resources and extend the supply of natural gas (however large it is) by using alternatives such as coal., even though coal plants produce higher cost power. Analyzing both alternatives permits calculation of the costs and other impacts of making the policy choice in this area.
6.3.2.3 Discount Rate / Cost of Capital
The discount rate is also the cost of capital, used to calculate the annual costs associated with a capital investment for a generating unit. Higher rates for cost of capital make high capital cost units less attractive. Higher discount rates make near-term costs more important than costs in later years. Our analysis uses 2005 constant dollars as the currency, meaning that inflation is not part of the determination of the cost of capital. Sound privately owned utilities in industrial countries have inflation-adjusted costs of capital (considering the weighted average of debt and equity) of about 8% per year or slightly below. Bangladesh currently is a riskier environment than a stable industrial economy, so our Base Case uses 12% discount rate. In the future, conditions may improve, leading to lower discount rates, or deteriorate, leading to higher discount rates.
The base case uses a 12% discount rate / cost of capital. We selected a higher cost of capital, 15%, to investigate the impact of a long-term, higher risk environment on the generation expansion plan. We also considered a lower value of 10%.
6.3.2.4 Unit Cost of Energy Not Served, $/KWH
The unit cost of ENS is the key input parameter associated with reliability. Its value is uncertain and difficult to measure. High values would lead to the need for more generating units and higher capital and operating costs. For our base case, we selected a value of $0.43 per KWH for ENS, consistent with what was used in the 1995 PSMP. We also considered values of $1.00 and $0.20, which we have used in other analyses. Because there is so much ENS today due to shortage of generation, and because there are fewer potential high-value industrial uses of electricity in Bangladesh, a range of values including much lower values than are used in developed countries seems appropriate. As with demand and discount rate, our analysis shows the impact of using different values for the unit cost of ENS. The unit cost of ENS is uncertain but is not a policy choice.
6.3.2.5 Use of LOLP Criterion
All the scenarios discussed above use a LOLP criterion while incorporating the cost of ENS into the economic objective function. This leaves the possibility that the LOLP criterion is governing (i.e., requires higher levels of reliability than the strictly economic approach would), meaning that the resource plan does not achieve the ideal least-cost balance of cost and reliability.
To investigate this possibility, we removed the LOLP criterion from the analysis and developed generation expansion plans using the same three values for the unit cost of ENS noted in the subsection above: $0.43 per KWH, $1.00 per KWH, and $0.20 per KWH. This permits evaluation of the impact of applying the LOLP criterion. The unit cost of ENS is uncertain, but whether the LOLP criterion should be applied is a policy choice.
6.3.3 Scenarios to Estimate Value
It can be useful to estimate the value of the energy and capacity provided by a generation expansion option. This is often applied where the cost of the option is uncertain, to set a limit on the cost that can be incurred; or where the option is a purchase, to set a limit on the amount that can be paid. This is accomplished by calculating the cost of replacing those services, which often can be easily done with the analytical tools used for generation
planning. This replacement cost is an upper limit on what should be paid (using least-cost principles) to construct and operate the option. The option need not be a power plant – it could be a DSM program, a capacity and energy purchase, a program to improve existing plants, or others.
The general approach is to insert a plant with similar operating characteristics to the option of interest, but with zero capital, fuel, and O&M costs. Compared to the Base Case, this “free” plant will reduce the need for other new units and will reduce capital, fuel, and O&M costs.
The amount of the overall cost reduction is the potential value to the system of the option of interest.
6.3.3.1 New Generating Plant
BPDB has power purchase agreements (PPAs) with IPP developers to purchase the output of IPP plants. It is of interest to know what the maximum value to BPDB is of the services provided by potential future IPPs. The same applies for any new plant of uncertain cost that BPDB may be considering building itself.
We used a 500 MW “free” power plant with the characteristics of a coal plant to investigate this issue. Because the fuel cost was zero, it dispatched to the extent of its availability.
Depending on the cost of its coal, an actual coal plant would not necessarily do that.
6.3.3.2 New Interconnection
Today Bangladesh has no electrical interconnections with other countries. There are many potential benefits to having substantial interconnections with utilities in neighboring countries.
- Reduction in planning reserves through pooling of loads and resources;
- Reduction in spinning and/or operating reserves through reducing the impact of the outage of the largest generator;
- Sharing of spinning and/or operating reserves;
- Access to the economies of scale of larger generating plants and in developing, constructing, and operating transmission systems;
- Reduced energy costs due to diversity of fuels and the time of peak demand; and
- Access to emergency support.
There is one key difficulty in including a new interconnection as an option for possible inclusion in the optimal generation expansion plan. Building an interconnection requires the agreement of and cooperation with the neighboring country. The amount and cost of the energy and/or capacity that the interconnection would permit to be imported also require the agreement of other parties. This introduces a strong element of uncertainty into the analysis. There are also problems with the costs of interconnections within the neighboring country.
Both countries need to see reasonable expectations of net benefits to their own systems. Overall benefits are likely to come from reserve sharing and energy exports.
We determine the value of a possible interconnection as follows. We assume that the interconnections with unspecified neighbors could provide 1,000 MW of capacity and 500 MW of energy around the clock, starting in 2009. We add a 500 MW “free” unit with zero
cost fuel that dispatches at nearly 100% capacity factor. At the same time we add another 500 MW plant with such high fuel costs and heat rate that it dispatches at nearly zero capacity factor. The energy and capacity from these units defer the need for new plant and reduce overall fuel costs because their energy is “free” and displaces other energy that has some cost. This reduces overall system costs, providing value for capacity and energy.
Reduction in system costs compared to the base case provides the value of the interconnections. This puts an upper limit on how much Bangladesh could spend to construct the interconnections and purchase the energy and capacity.
Table 6-2 summarizes the scenarios studied using production simulation and system optimization.
Section 6 Generation Expansion Plan
Table 6-2 Scenarios Evaluated
Case No. | Objective | Demand | Gas Supply | Gas Price | COENS (Note 1) $/kWh | LOLP Criterion Applied | Discount Rate | Other New Plants | Interconnection |
Production Simulation / System Optimization Analysis - Scenarios to Evaluate the Impact of Changes to Base Case Conditions |
1 | Establish Base Case | Base = Medium | Base = sufficient gas | $3.02/GJ (Note 2) | Base = 0.43 | Yes | Base = 12% real | Base = none | Base = None |
2 | High demand | High | Base | Base | Base | Base | Base | Base | Base |
3 | Low demand | Low | Base | Base | Base | Base | Base | Base | Base |
4 | Limited Gas | Base | Exisitng fields only | Base | Base | Base | Base | 10,000 MW of Coal | Base |
5 | Limited Gas - Early Coal | Base | Exisitng fields only | Base | Base | Base | Base | Coal Units Come Sooner | Base |
6 | Fuel Security | Base | Base | Base | Base | Base | Base | 4,000 MW of Dom Coal | Base |
7 | High Unit COENS | Base | Base | Base | 1.00 | Base | Base | Base | Base |
8 | Low Unit COENS | Base | Base | Base | 0.20 | Base | Base | Base | Base |
9 | Lower discount rate | Base | Base | Base | Base | Base | 10% | Base | Base |
10 | Higher discount rate | Base | Base | Base | Base | Base | 15% | Base | Base |
11 | No LOLP criterion applied - Base COENS | Base | Base | Base | 0.43 | No | Base | Base | Base |
12 | No LOLP criterion applied - High COENS | Base | Base | Base | 1.00 | No | Base | Base | Base |
13 | No LOLP criterion applied - Low COENS | Base | Base | Base | 0.20 | No | Base | Base | Base |
Production Simulation / System Optimization Analysis - Scenarios to Estimate Value |
14 | Determine value of 500 MW plant | Base | Base | Base | Base | Base | Base | Add 500 MW "free" plant | Base |
15 | Determine value of inter- connection capacity and energy | Base | Base | Base | Base | Base | Base | Base | Add 1,000 MW "free" capac at 50% capac fac |
| | | | | | | | | |
Note 1. COENS = Unit Cost of Energy Not Served | Note 2. $3.02 = 75% of levelized fuel oil cost | |
6.4 SCREENING CURVE ANALYSIS
6.4.1 Description of Screening Curve Analysis
The screening curve method combines simplified representations of generation costs and system load projections to approximate the optimum mix of generating technologies. The basic approach is to construct cost curves for each technology and then to match the points of intersection with corresponding load points to determine the competitiveness of each technology and the most cost-effective operating regimes and capacities for each technology. The technique captures the major tradeoffs between capital costs, operating costs, and level of use for proposed types of new generating units. This method recognizes, for example, that the low capital/lower efficiency characteristics of combustion turbines are preferable to high capital/higher efficiency characteristics of combined cycle units for applications requiring small amounts of annual generation. Most important, this method requires only minimal technical and analytical inputs while it quickly eliminates very uncompetitive technologies and provides simplified estimates of optimal technology mixes.
It is also important to be aware of the limitations associated with screening curves. Screening curve analysis is not an adequate substitute for detailed production cost or expansion planning analysis. Important factors, such as forced outages, unit sizes, and system reliability are not treated directly with screening curves.
The screening curve method expresses the total annual energy production cost for a generating unit, including all capital-related and operating expenses, as a function of the capacity factor. The following equation defines how the cost curves are developed for this approach:
Total cost = (annualized fixed costs) + (variable cost x capacity factor x hours per year).
Figure 6-1 presents this equation graphically with fixed costs represented by the vertical axis intercept and variable costs shown as the slope of the line. Fixed costs are annualized capital- related costs and annual O&M costs that do not change with the annual KWH production of the unit. For example, if the unit cost to build one KW of generating capacity is $500, and the plant's life is 30 years, and a constant capital charge of $60 per KW-year is sufficient to recover all capital-related costs (including depreciation, return, taxes, and insurance) on a net present value basis, then the levelized fixed charge rate is 0.12 (60/500). Fixed O&M costs are expressed in $/KW-year and are the same at all capacity factors. So, as an example, if fixed O&M cost is 7 $/KW-year then stating point, or intercept with vertical axis would be at 67 $/KW.
Variable costs are fuel costs and variable O&M costs that do vary with the KWH output of the unit, such as consumables. Typically, the variable costs expressed in $/KWH are known or can be calculated. The variable cost component of total annual energy product cost at a given capacity factor is the variable cost in $/KWH times the hours per year of operation.
The capacity factor times 8,760, the number of hours in the year, gives the hours per year of operation. If we continue with our numerical example, if fuel and variable O&M costs add up to 30 $/MWH or 3 cents/KWH, then for each KWH produced costs go up 3 cents. We calculated costs for the range of capacity factors up to 90%, not 100%, because planned and unplanned maintenance make it extremely difficult to exceed 90% capacity factor on a regular basis.
Figure 6-1 Cost Representation for Screening Analysis Method
6.4.2 Cases and Input Data
We developed six sets of screening curves, each intended to address a specific issue. Cases that were evaluated are:
6.4.2.1 Base Load Technologies
We compared technologies best suited for base load (low capacity factor) duty. We considered a range of unit sizes for some of the technologies as an indication of how unit size for a given technology affects the cost of generation. This analysis also provides direction of what unit sizes should be used for technology comparison. We made comparison for three unit sizes for CC units, two for coal-fueled steam, and two for natural gas fueled steam.
6.4.2.2 Peaking Technologies
We compared technologies best suited for peaking (low capacity factor) duty. We considered two unit sizes for SCGT as an indication of how unit size affects their cost of generation.
6.4.2.3 Cost of ENS
At low capacity factors, the screening curves for the different technologies have high unit costs expressed in $/MWH. All the annual fixed costs are spread over few MWH. The cost of ENS has no annual fixed cost, so for very low capacity factors not serving load can be less costly than building new units to operate in that range. We emphasize that production simulation runs are needed to determine on a probabilistic basis the operating capacity factors of individual units.
6.4.2.4 All Technologies and Least Cost Curve
Technology analysis provides first direct comparison for all competitive technology options, using the lowest cost unit sizes. This provides the overall least cost curve. The least cost curve is the set of line segments that are the lowest cost alternative at each capacity factor.
6.4.2.5 Natural Gas Price
The Base Case analysis uses a natural gas price of $3.02/GJ, corresponding to 75% of the forecast of the levelized price of fuel oil. As noted in Section 4, this is well above the current price charged BPDB for natural gas of 73.91 Taka/1,000 cubic feet, equivalent to about
$1.25/GJ. Prices other than the Base Case value are clearly possible, especially lower prices. To evaluate the impact of a range of fuel prices, we performed screening analysis using values of $3.02/GJ (Base), $1.25/GJ (Low), and $4.03/GJ (High). The High value corresponds to 100% of the forecast of the levelized price of fuel oil. We did not conduct production simulation / system optimization on prices other than the Base Case price
6.4.2.6 Breakeven Price of Coal
We calculated the price of coal for which the annual costs are the same for the coal-fueled 500 MW steam unit and the natural gas-fueled CC unit. This gives a measure of how low coal costs would have to be to make the cost of power for a steam plant competitive for base load duty.
Table 6-3 summarizes the input data for the options subjected to screening analysis.
Table 6-3 Screening Analysis Options
Unit Type | Net Unit Capacity (At Grid) | Fuel | Levelized Fuel Prices, $/million kcal | Total Project Cost $/kW | Plant Life, Years | Net Full Load Heat Rate At Grid, kcal/KWH | Weeks/year Sched Maint | Fixed O&M Coat, $/kW- month | Variable O&M Cost, $/MWH |
Steam | 300 | Dom Coal | 7.97 | 1,366 | 30 | 2,173 | 8 | 0.58 | 1.80 |
Steam | 500 | Dom Coal | 5.86, 7.97 | 1,111 | 30 | 2,154 | 8 | 0.58 | 1.80 |
CCGT | 300 | Gas | 12.65 | 670 | 25 | 1,720 | 6 | 0.42 | 2.00 |
CCGT | 450 | Gas | 4.96, 12.65, 16.86 | 593 | 25 | 1,686 | 6 | 0.38 | 1.80 |
CCGT | 700 | Gas | 4.96, 12.65, 16.86 | 502 | 25 | 1,564 | 6 | 0.38 | 1.80 |
SCGT | 100 | Gas | 4.96, 12.65, 16.86 | 401 | 20 | 2,687 | 4 | 0.42 | 2.50 |
SCGT | 150 | Gas | 4.96, 12.65, 16.86 | 349 | 20 | 2,605 | 4 | 0.42 | 2.50 |
Steam | 300 | Gas | 12.65 | 974 | 30 | 2,127 | 6 | 0.58 | 1.60 |
Steam | 500 | Gas | 12.65 | 789 | 30 | 2,109 | 6 | 0.58 | 1.60 |
Nuclear | 500 | Nuclear | 2.09 | 2,717 | 40 | 2,598 | 6 | 1.67 | 0.50 |
Diesel | 10 | Diesel | 25.85 | 450 | 15 | 2,900 | 3 | 0.83 | 3.00 |
| | | $/KWH | | | | | | |
COENS | | | 0.20, 0.43, 1.00 | | | | | | |
6.4.3 Results
6.4.3.1 Base Load Technologies
Figure 6-2 compares the base load technologies. It shows that:
- In every case, larger unit size reduces the annual costs. This result was expected since the larger size units in each set all have lower capital cost per KW and higher efficiency than the other units in the set.
- All combined cycle units are lower in cost than steam units.
- Using natural gas in steam units results in higher cost that using in combined cycles, or using coal-fueled steam units for capacity factors above about 30%.
Figure 6-2 Base Load Technologies
6.4.3.2 Peaking Technologies
Figure 6-3 compares the peaking technologies. It shows that:
- For the SCGT, larger unit size reduces the annual costs.
- The diesel is not competitive at any capacity factor. However, its small size makes it more attractive for applications where the unit serves a small load.
Figure 6-3 Peaking Technologies
For both base load and peaking technologies, larger unit sizes are lower in costs. Despite this, several reasons not incorporated in screening analysis might cause a utility to select a smaller unit size. The main factors for Bangladesh are perceived risk and relative size compared to annual load growth. Annual load growth in the Base Case exceeds 450 MW by 2010, but does not surpass 700 MW until 2015. The largest CC that Bangladesh has experience with is 450 MW. The 700 MW CC is viewed as riskier technically, financially, and from the point of view of obtaining approvals. Accordingly, we did not permit the first 700 MW CC to begin operation until 2018. Until then the 450 MW CC was the lowest cost CC option.
6.4.3.3 Cost of ENS
Figure 6-4 compares the least cost peaking technologies with the COENS lines. Note that the scale on the horizontal axis has changed. It is zero to 9% instead of zero to 90%, in order to provide more detail in the region of interest. It shows that:
- At lower values of ENS, the breakeven point with the SCGT moves to higher capacity factors.
- The breakeven capacity factors range from less than 1% to about 3.5%.
Figure 6-4 Peaking Technologies vs. COENS
6.4.3.4 All Technologies and Least Cost Curve
Figure 6-5 compares all technologies and presents the least cost curve. The technologies that were not competitive in the base load or peaking comparisons are still uncompetitive, of course. The least cost curve consists of ENS for capacity factors from zero to about 2% , the 150 MW SCGT from 2% to about 15%, and the 700 MW CC above 15%. For the first few years we assume the 700 MW CC will not be used. During that time the least cost curve includes the 150 MW SCGT from 2% to about 25% capacity factors and the 450 MW CC above 25%. The steam technologies and the diesel unit are not very close to competitive at any capacity factor.
Figure 6-5 All Technologies and Least Cost Curve
Capacity factor has a different meaning for the ENS line than the other lines. Using the 150 MW SCGT as an example, it has a least cost capacity factor range 2% to 25% if the 700 MW CC will not be used. In a system as large as Bangladesh’s, many such units would be installed. Ideally each would dispatch in that range. If small differences in parameters such as heat rate, gas cost, or others caused differences in dispatch order, the most-used unit should have a capacity factor close to 25% and the least used one close to 2%. Even if all dispatched equally, it would be possible to calculate a capacity factor for each.
For ENS capacity factor is not precisely defined because ENS has no specific “capacity” similar to that of a SCGT. The calculation of the ENS line was equivalent to assuming a zero capital cost, zero O&M cost “unit” whose cost was the unit cost of ENS times the hours per year corresponding to the capacity factors shown. It would be extremely unusual for ENS to occur in that pattern. As an illustration, in a year with 1,000 hours of ENS the largest inability to serve load might be 50 MW for one hour. Smaller outages would occur more frequently such that the sum of the products of outage MW times hours of occurrence would equal 1,000 MWH. It’s more useful to think of the lower breakeven capacity factor for the
SCGT as a minimum capacity factor. If the next SCGT to be added to the generation resource plan cannot achieve a capacity factor of at least 2%, it probably should not be built. Only probabilistic production simulation can estimate how much ENS the next SCGT would eliminate and what its capacity factor would be.
6.4.3.5 Natural Gas Price
Figures 6-6 and 6-7 show the impact of higher and lower natural gas prices on the least cost line.
Figure 6-6 Higher Gas Price
Figure 6-7 Lower Gas Price
In Figure 6-6, the higher levelized gas price of $4.03/GJ vs. $3.02/GJ in the Base Case causes the combined cycles and even the 500 MW coal plants to be more competitive. The SCGT has a smaller portion of the least cost curve. In contrast, the lower levelized gas price of
$1.25/GJ greatly extends the SCGTs portion of the least cost curve, with significant implications.
Section 8.1.1.3, Constraints and Other Issues, discusses the impact of gas price on planning in more detail.
6.4.3.6 Breakeven Price of Coal
Figure 6-8 shows the impact of using a coal price ($1.46/GJ vs. $1.90/GJ in the Base Case) that produces the same annual cost as the 450 MW CC at a capacity factor of 80%. This provides a measure of the maximum price of coal that would permit coal to be a least-cost option. Probably the coal price would have to be even lower than that. The 700 MW CC is still lower in cost, and the comparison is based on a levelized natural gas price of $3.02/GJ, well above today’s price. The GOB might be willing to pay a premium to diversify its fuel sources and extend the life of its gas resource, but how large a premium (if any) is a policy matter. Whether a price of $1.46/GJ might reasonably be achieved in Bangladesh is not known. There is considerable uncertainty in the Base Case price estimate, so prices well below the Base Case values may well be feasible.
Figure 6-8 Breakeven Coal Price
6.4.4 Summary of Conclusions
From the screening analysis, we can draw several important conclusions:
- In every case, larger unit size reduces the annual costs. This result was expected since the larger size units in each set all have lower capital cost per KW and higher efficiency than the other units in the set.
- All combined cycle units are lower in cost than steam units.
- Using natural gas in steam units results in higher costs that using it in combined cycles, and higher than the costs from coal-fueled steam units for capacity factors above about 30%.
- The least cost curve consists of ENS for capacity factors from zero to about 2% , the 150 MW SCGT from 2% to about 15%, and the 700 MW CC above 15%. For the first few years we assume the 700 MW CC will not be used. During that time the least cost curve includes the 150 MW SCGT from 2% to about 25% capacity factors and the 450 MW CC above 25%.
- The steam technologies and the diesel unit are not very close to competitive at any capacity factor.
- Depending on assumptions regarding the unit cost of ENS, the minimum operating capacity factor for SCGT should be from 1% to 4%.
- For coal to become a least cost option, the price of coal would have to be $1.46/GJ or below. The GOB might be willing to pay a premium to diversify its fuel sources and extend the life of its gas resource, but how large a premium (if any) is a policy matter.
- Using the higher levelized gas price of $4.03/GJ vs. $3.02/GJ in the Base Case causes the combined cycles and even the 500 MW coal plants to be more competitive. The SCGT has a smaller portion of the least cost curve. In contrast, the lower levelized gas price of $1.25/GJ greatly extends the SCGTs portion of the least cost curve, with significant implications.
6.5 GENERATION EXPANSION PLAN OVERVIEW
6.5.1 Electric Generation Production Simulation and Optimization
The purpose of electric power system generation production simulation is to simulate the operation of generating units and calculate the expected amount of energy generated by each unit to meet projected loads. The program uses a load duration curve simulation method to dispatch resources to meets the demand for electricity described by the input peak and energy demand for each time period. The model takes into account both planned resource variations (periods of scheduled plant outages) and unplanned resource variations (such as the effect of plant forced outages).
It determines the least-cost manner of operating generating units within a given period subject to constraints, such as unit minimum load levels and spinning reserve requirements. The generation simulation module also calculates a wide range of results such as reliability statistics, fuel use, and production costs.
Production simulation is fundamental to the process of system optimization. The objective of generation planning analysis is to provide for the generation expansion of a power system to adequately meet the demand for electricity at minimum cost.
The generation expansion optimization algorithm uses information from the simulation analysis to make decisions of the optimal type, size, and timing of plant additions. Expansion planning is essentially a series of production cost runs. The optimization program uses
annual production cost information to determine the costs and benefits of adding additional resources during the whole planning period. These are elements of the optimization function used to determine the most cost-effective mix of resource options. The costs of a generation plan are measured by the discounted present value of overall system costs, including the costs of not meeting demand.
The goal of the optimization process is to minimize the present value of all generation-related system costs over the planning period. In simplistic terms, adding or deleting a resource from an existing plan provides both costs and benefits. For example, adding a new plant to the resource mix reduces fuel and O&M costs from other plants, and reduces the costs associated with ENS. It adds capital, O&M, and fuel costs for the added plant. When the cost reductions are large enough to offset the operating and investment-related costs of the new plant, the new plant may be added to the resource plan.
6.5.2 WASP-IV Electricity System Simulation and Optimization
The Wien Automatic System Planning Package (WASP) was originally developed by the Tennessee Valley Authority (TVA) and Oak Ridge National Laboratory (ORNL) of the United States of America to meet the needs of the International Atomic Energy Agency’s (IAEA's) Market Survey for Nuclear Power in Developing Countries conducted by the Agency in 1972-1973.
Based on the experience gained in using the program, IAEA developed improved versions of WASP in 1976 (WASP-II) and 1980 (WASP-III). Development of a new version was initiated in 1992 and led to the completion of the current version, WASP-IV.
Like its predecessor, WASP-IV is designed to find the economically optimal generation expansion policy for an electric utility system within user-specified constraints. It utilizes probabilistic estimation of system production costs, energy not served (unserved energy) and its cost, and system reliability. Its analytic approach incorporates linear programming techniques for determining the optimal dispatch policy satisfying constraints on environmental emissions, fuel availability, and electricity generation by some plants, and dynamic techniques for optimization by comparing the costs of alternative system expansion policies.
The WASP-IV code permits finding the optimal expansion plan for a power generating system over a period of up to thirty years, within constraints given by the planner. The optimum is evaluated in terms of minimum discounted total costs. Each possible sequence of power units added to the system meeting the constraints is evaluated by means of a cost function (the objective function) that is composed of:
- Capital investment costs including salvage value.
- Fuel and fuel inventory costs
- Non-fuel operation and maintenance costs
- Cost of the energy not served
The optimal expansion plan is the plan with the lowest sum of the yearly present worth of all the cost categories.
The most significant inputs to the production simulation part of the model are the overall level and pattern of energy demands and the operating cost and performance characteristics of existing and potential new generating units, including thermal, hydroelectric, and pumped storage. For system optimization other information, such as capital costs, the unit cost of energy-not-served, and discount rate is needed.
6.5.3 Data Review and Inputs
We summarize below the kinds of key data needed as input to the WASP-IV program for analysis of the Bangladeshi generation system.
- Annual peak demand in MW, annual energy demand in GWH, and other data related to demand as presented in Section 3.
- Fuel availability and price, as presented in Section 4.
- Performance and cost of the units of the existing generation system, as presented in Section 2.
- Performance and cost of the potential new units or other additions (e.g., purchases), as presented in Section 5.
- Other parameters primarily related to reliability or financial matters, including:
− Discount rate: Base Case is 12% real.
− Unit cost of ENS: Base Case is US$0.43/KWH.
− Minimum LOLP percentage: Base Case is 1%.
− Escalation of cost components: Base Case assumed no escalation and uses values expressed in 2005 US$.
6.6 GENERATION EXPANSION PLAN RESULTS
This section presents generation planning results for the Base Case scenario, followed by results for the other scenarios.
The screening analysis indicated that the likely units to enter the least cost plan would be 150 MW SCGT, 450 MW CC (until 2018), and 700 MW CC. Initial test WASP-IV runs confirmed that this in fact was the case. For more detailed analysis in most cases we limited the choices of new units to those three unit types. We relaxed that limitation only to study specific scenarios where another unit type was the focus of the study.
6.6.1 Base Case
6.6.1.1 Unit Additions and System Reliability
Table 6-4 presents generation additions for the Base Case. New unit additions consist of a mixture of 450 MW and 700 MW CC, and 150 MW SCGT. Table 6-4 shows only the new units added by the program as part of a least cost plan. We do not show as additions the capacity from existing units, or committed units that will start operation in 2005 or thereafter. The capacity from those categories of units, and the impact of retirements, is included in the totals shown in the column labeled “Installed Capacity, MW”.
Combined cycles dominate system additions in terms of MW. As soon as we allow 700 MW units as options, no more 450 MW units appear.
Our lead time assumptions are 24 months for the SCGT and 36 months for the CC. Thus the earliest full year that SCGTs could appear is 2008, and the earliest that CCs could appear is 2009. We did not apply the LOLP criterion limiting LOLP to a maximum of 1% in 2005 – 2008, meaning that in 2008 units were added only for economic reasons. In 2009 and thereafter both SCGT and CC could be added.
Table 6-4 Base Case Unit Additions and System Reliability Indices
| | Unit Additions, Number of Units | | System Reliabilty Indices |
Year | Peak Load, MW | 700 MW CC | 450 MW CC | 150 MW SCGT | Installed Capacity, MW | LOLP, % | ENS, GWH | Reserve Margin, % |
2005 | 4,308 | 0 | 0 | 0 | 4,458 | 8.138 | 180.8 | 3% |
2006 | 4,693 | 0 | 0 | 0 | 4,683 | 10.884 | 320.9 | 0% |
2007 | 5,112 | 0 | 0 | 0 | 5,425 | 6.350 | 137.5 | 6% |
2008 | 5,569 | 0 | 0 | 2 | 6,002 | 5.135 | 108.3 | 8% |
2009 | 6,066 | 0 | 1 | 0 | 7,313 | 0.845 | 8.9 | 21% |
2010 | 6,608 | 0 | 2 | 0 | 7,986 | 0.750 | 8.2 | 21% |
2011 | 7,148 | 0 | 1 | 1 | 8,586 | 0.797 | 9.0 | 20% |
2012 | 7,732 | 0 | 2 | 0 | 9,449 | 0.490 | 5.1 | 22% |
2013 | 8,364 | 0 | 1 | 1 | 9,979 | 0.834 | 10.1 | 19% |
2014 | 9,047 | 0 | 2 | 0 | 10,879 | 0.654 | 7.4 | 20% |
2015 | 9,786 | 0 | 2 | 1 | 11,579 | 0.937 | 12.6 | 18% |
2016 | 10,512 | 0 | 2 | 0 | 12,479 | 0.848 | 11.2 | 19% |
2017 | 11,291 | 0 | 0 | 5 | 13,229 | 0.997 | 13.5 | 17% |
2018 | 12,128 | 1 | 0 | 2 | 14,229 | 0.912 | 12.2 | 17% |
2019 | 13,027 | 1 | 0 | 3 | 15,243 | 0.880 | 11.9 | 17% |
2020 | 13,993 | 2 | 0 | 0 | 16,643 | 0.578 | 6.7 | 19% |
2021 | 14,924 | 1 | 0 | 3 | 17,455 | 0.816 | 11.2 | 17% |
2022 | 15,917 | 2 | 0 | 0 | 18,526 | 0.949 | 15.6 | 16% |
2023 | 16,977 | 2 | 0 | 1 | 19,867 | 0.811 | 12.5 | 17% |
2024 | 18,107 | 2 | 0 | 0 | 21,070 | 0.923 | 15.9 | 16% |
2025 | 19,312 | 1 | 0 | 4 | 22,370 | 0.950 | 16.1 | 16% |
| Total | 12 | 13 | 23 | 48 | | | |
| Total MW | 8,400 | 5,850 | 3,450 | 17,700 | | | |
| Percent | 47% | 33% | 19% | ^ Total Units and MW Added | |
Table 6-4 also shows three reliability indices: LOLP, ENS, and reserve margin. Reserve margin is defined as follows:
(Installed Capacity – Peak Load) / Peak Load
Reliability is much lower in 2005 – 2007 because there is not enough time to install any units other than the committed units under way. In 2008 new SCGT can be added, but the LOLP reliability criterion is not applies. Reliability improves, but only as required by economics.
As soon as the LOLP reliability criterion is applied and there is enough time to build new units, in 2009, system reliability meets the criterion and all measures of reliability stay
roughly constant over the rest of the planning period. There is a slight trend of declining reserve margin over that period, and of increasing ENS. Both are to be expected as the system grows larger.
6.6.1.2 Costs
Table 6-5 provides a summary for system operating, investment, and energy-not-served costs. We call the sum of operating and investment costs “Total Direct Costs”, because they are the costs seem by the utility. When ENS costs are added, we call the result “Total Costs” because they include the impact on customers of the loss of electric service some of the time.
The cost summary is the key indicator used for comparing cost effectiveness of different generation development scenarios. More specifically, it is the Net Present Value (NPV) referred to the year 2005 that takes into account when costs occur in time and brings all costs to the base year. For each category of cost, NPV is the sum of the present values referred to the beginning of 2005 of each year’s costs. The NPV is used to determine the optimal development scenario or to compare different development options.
Table 6-5 Base Case System Costs
| All Costs in Millions of 2005 US$ |
Year | Operating Costs | Capital Costs | Total Direct Costs | ENS Costs | Total Costs |
Fixed & Variable O&M | Fuel |
2005 | 124 | 702 | 0 | 826 | 78 | 904 |
2006 | 138 | 738 | 63 | 938 | 138 | 1,076 |
2007 | 157 | 811 | 305 | 1,273 | 59 | 1,332 |
2008 | 161 | 867 | 478 | 1,506 | 47 | 1,552 |
2009 | 180 | 924 | 467 | 1,570 | 4 | 1,574 |
2010 | 185 | 964 | 518 | 1,667 | 4 | 1,670 |
2011 | 193 | 1,036 | 467 | 1,696 | 4 | 1,700 |
2012 | 202 | 1,101 | 546 | 1,849 | 2 | 1,851 |
2013 | 210 | 1,177 | 636 | 2,023 | 4 | 2,028 |
2014 | 220 | 1,258 | 604 | 2,082 | 3 | 2,085 |
2015 | 232 | 1,347 | 349 | 1,928 | 5 | 1,933 |
2016 | 243 | 1,434 | 494 | 2,170 | 5 | 2,175 |
2017 | 256 | 1,569 | 576 | 2,401 | 6 | 2,407 |
2018 | 269 | 1,678 | 750 | 2,697 | 5 | 2,703 |
2019 | 273 | 1,583 | 645 | 2,501 | 5 | 2,506 |
2020 | 288 | 1,683 | 787 | 2,758 | 3 | 2,761 |
2021 | 294 | 1,767 | 832 | 2,893 | 5 | 2,898 |
2022 | 304 | 1,862 | 819 | 2,984 | 7 | 2,991 |
2023 | 316 | 1,968 | 589 | 2,874 | 5 | 2,879 |
2024 | 328 | 2,080 | 309 | 2,717 | 7 | 2,724 |
2025 | 346 | 2,210 | 0 | 2,556 | 7 | 2,563 |
Total | 4,916 | 28,758 | 10,236 | 43,910 | 402 | 44,313 |
Total NPV | 1,469 | 8,268 | 3,042 | 12,779 | 270 | 13,050 |
Fixed and variable O&M costs represent costs for operating and providing regular maintenance for all generating units. Fuel cost gives total cost for fuel expenditures in all power plants, taking into account how each unit operates in the system. The sum of O&M and fuel costs is the total operating cost.
Capital costs represent the cost for building new units. WASP-IV spreads the total cost of a new unit over the input construction times for the different kinds of units. WASP-IV calculates the interest during construction (IDC) that applies each year, based on the discount rate. The values shown in Table 6-5 are the yearly values including IDC. The program assumes that a new unit is available for use for the entire year it is commissioned, effectively starting the first day of the year. With a two year construction time, the earliest year that a new SCGT can be available by the first day of the year is 2008. Thus for the two SCGT units coming on line in 2008, all expenditures occur in 2006 and 2007, and none in 2005. The expenditures for the units coming on line in 2025 occur in the two or three preceding years, with none coming in 2025. The last year of the study period is 2025, so no new units are under construction in 2022 – 2025 to cater for years after 2025. Therefore there are no capital costs in that year, and declining capital costs in 2022, 2023, and 2024.
We also show the cost of ENS, since this number is used for scenario comparison, but it is not a direct cost to the utility. Note that only in 2005 – 2008 is the cost of ENS a major part of overall cost. This is typical of well-balanced utility systems.
There are many other utility cost that are incurred in operating the generation system, such as general and administrative costs and depreciation of existing assets that are not included in our economic analysis. These costs have to be included when analyzing utility financials and tariffs, but they are the same for all scenarios and do not have an impact on our scenario comparison and ranking.
6.6.1.3 Fuel Requirements
Fuel requirement numbers are used for near- and long-term fuel supply planning. Fuel supply planning is critical in assuring that the correct type and the amount of fuel is available to reliably operate the power system.
Figure 6-9 presents Base Case fuel requirements for major fuel sources. Fuel oil, diesel oil, and coal use are expressed in million metric tones and plotted against the left vertical axis, while natural gas is presented in billion SCF and plotted against the right vertical axis. Table 6-7 provides the same information in tabular form. Since all new units use natural gas as fuel, there is a clear trend of using much more natural gas over time. At the same time natural gas by 2019 replaces all liquid fuels. The Barapukuria coal plant provides some domestic coal use.
In its routine reports, WASP-IV tabulates fuel use in money terms, not in physical terms. Table 6-6 shows the conversion factors we used to calculate fuel use in physical quantities.
Table 6-6 Conversion Factors in Calculations of Fuel Usage
Fuel | Price, $/GJ | Heat Content |
Natural Gas | 3.02 | 950 KJ/SCF |
Domestic Coal | 1.90 | 23 GJ/metric tonne |
Imported Coal | 2.19 | 23 GJ/metric tonne |
Fuel Oil | 4.03 | 41 GJ/metric tonne |
Diesel Oil | 6.18 | 43 GJ/metric tonne |
Figure 6-9 Base Case Fuel Use
Table 6-7 Base Case Fuel Use
Year | Gas, Billion Cubic Ft | Fuel Use in Thousand Metric Tonnes |
Domestic Coal | Imported Coal | Fuel Oil | HS Diesel |
2005 | 225 | 0 | 0 | 274 | 42 |
2006 | 231 | 327 | 0 | 281 | 55 |
2007 | 255 | 644 | 0 | 262 | 32 |
2008 | 277 | 635 | 0 | 248 | 5 |
2009 | 304 | 569 | 0 | 163 | 0 |
2010 | 318 | 530 | 0 | 161 | 0 |
2011 | 344 | 523 | 0 | 162 | 0 |
2012 | 367 | 507 | 0 | 154 | 0 |
2013 | 393 | 509 | 0 | 161 | 0 |
2014 | 422 | 500 | 0 | 159 | 0 |
2015 | 457 | 498 | 0 | 84 | 0 |
2016 | 487 | 496 | 0 | 82 | 0 |
2017 | 534 | 498 | 0 | 83 | 0 |
2018 | 572 | 496 | 0 | 84 | 0 |
2019 | 543 | 525 | 0 | 0 | 0 |
2020 | 579 | 512 | 0 | 0 | 0 |
2021 | 607 | 519 | 0 | 0 | 0 |
2022 | 641 | 507 | 0 | 0 | 0 |
2023 | 678 | 503 | 0 | 0 | 0 |
2024 | 717 | 498 | 0 | 0 | 0 |
2025 | 762 | 503 | 0 | 0 | 0 |
Total | 9,713 | 10,297 | 0 | 2,355 | 134 |
6.6.1.4 Power Plant Sites
Table 6-8 lists the sites selected for the Base Case resource plan. It includes both committed and the new units determined by the WASP analysis. We placed the units at sites following the approach outlined in Section 5.
After the Base Case set of system additions was developed, BPDB determined that one of the 450 MW combined cycles identified for 2010 should be replaced with three smaller units: two additional 150 MW combined cycles in 2008 and one additional 100 MW simple cycle gas turbine in 2009. This treats these units in effect as committed units. Table 6-8 reflects that change.
Table 6-8 Sites and Capacity Addition
Plant (Com = Committed) | Region | Unit Type | Unit # | Actual Year of Operation | Fuel | Net Capac, MW |
Gorasal Unit 1 Com (Under Maintenance) | Dhaka | ST | 1 | 2005 | Gas | 37 |
Tongi Com | Dhaka | CT | 1 | 2005 | Gas | 104 |
Sidhirganj Com | Dhaka | ST | 1 | 2005 | Gas | 197 |
Mymenshing RPC Com (CC Conv) | Central | ST/CC | 1 | 2006 | Gas | 70 |
Barapukuria Coal Com | Northern | ST | 1 | 2006 | Dom Coal | 115 |
Barapukuria Coal Com | Northern | ST | 2 | 2006 | Dom Coal | 115 |
Baghabari Barge Mtd Com (CC Conv) | Northern | ST/CC | 1 | 2006 | Gas | 40 |
Sidhirganj Com | Dhaka | CT | 1 | 2007 | Gas | 119 |
Sidhirganj Com | Dhaka | CT | 2 | 2007 | Gas | 119 |
Sidhirganj Com | Dhaka | CT | 3 | 2007 | Gas | 119 |
Sylhet Com | Central | CT | 1 | 2007 | Gas | 99 |
Chandpur Com | Southern | CC | 1 | 2007 | Gas | 99 |
Baghabari Barge Mtd Com | Northern | CC | 1 | 2007 | Gas | 130 |
Fenchuganj Com | Central | CC | 1 | 2008 | Gas | 88 |
Meghnaghat Com | Dhaka | CC | 1 | 2008 | Gas | 450 |
Sidhirganj Com | Dhaka | ST | 2 | 2009 | Gas | 197 |
Karnafuli Hydro HY2 Com | Southern | HY | 1 | 2009 | Hydro | 100 |
Khulna ST#2 Com | Western | ST | 2 | 2009 | Gas | 197 |
Siraganj Com | Northern | CC | 1 | 2009 | Gas | 450 |
Haripur | Dhaka | CC | 1 | 2008 | Gas | 150 |
Sikalbaha | Southern | CT | 1 | 2008 | Gas | 150 |
Bogra | Northern | CT | 1 | 2008 | Gas | 150 |
Bhola | Western | CC | 1 | 2008 | Gas | 150 |
Meghnaghat | Dhaka | CC | 2 | 2009 | Gas | 450 |
Khulna | Western | CT | 1 | 2009 | Gas | 100 |
Sikalbaha | Southern | CC | 1 | 2010 | Gas | 450 |
Meghnaghat | Dhaka | CC | 3 | 2011 | Gas | 450 |
Sylhet | Central | CT | 1 | 2011 | Gas | 150 |
Sirajganj | Northern | CC | 2 | 2012 | Gas | 450 |
Bheramara | Western | CC | 1 | 2012 | Gas | 450 |
Haripur | Dhaka | CT | 1 | 2013 | Gas | 150 |
Madanhat/New Sikalbaha | Southern | CC | 1 | 2013 | Gas | 450 |
Amibazar/Dhaka West | Dhaka | CC | 1 | 2014 | Gas | 450 |
Madanhat/New Sikalbaha | Southern | CC | 2 | 2014 | Gas | 450 |
Siddhirganj | Dhaka | CC | 1 | 2015 | Gas | 450 |
Shahjbazar | Central | CT | 1 | 2015 | Gas | 150 |
Khulna | Western | CC | 1 | 2015 | Gas | 450 |
Amibazar/Dhaka West | Dhaka | CC | 2 | 2016 | Gas | 450 |
Rajshanj | Northern | CC | 1 | 2016 | Gas | 450 |
Ashuganj | Dhaka | CT | 1 | 2017 | Gas | 150 |
Amibazar/Dhaka West | Dhaka | CT | 1 | 2017 | Gas | 150 |
Sylhet | Central | CT | 2 | 2017 | Gas | 150 |
Mymensingh New Site | Central | CT | 1 | 2017 | Gas | 150 |
Madanhat/New Sikalbaha | Southern | CT | 1 | 2017 | Gas | 150 |
Meghnaghat New Site | Dhaka | CC | 1 | 2018 | Gas | 700 |
Plant (Com = Committed) | Region | Unit Type | Unit # | Actual Year of Operation | Fuel | Net Capac, MW |
Madanhat/New Sikalbaha | Southern | CT | 2 | 2018 | Gas | 150 |
Saidpur | Northern | CT | 1 | 2018 | Gas | 150 |
Mawa | Dhaka | CC | 1 | 2019 | Gas | 700 |
Fenchuganj | Central | CT | 1 | 2019 | Gas | 150 |
Mymensingh New Site | Central | CT | 2 | 2019 | Gas | 150 |
Feni | Southern | CT | 1 | 2019 | Gas | 150 |
Meghnaghat New Site | Dhaka | CC | 2 | 2020 | Gas | 700 |
Madanhat/New Sikalbaha | Southern | CC | 1 | 2020 | Gas | 700 |
Amibazar/Dhaka West | Dhaka | CT | 2 | 2021 | Gas | 150 |
Mawa | Dhaka | CC | 2 | 2021 | Gas | 700 |
Baghabari | Northern | CT | 1 | 2021 | Gas | 150 |
Barisal | Western | CT | 1 | 2021 | Gas | 150 |
Mawa | Dhaka | CC | 3 | 2022 | Gas | 700 |
Madanhat/New Sikalbaha | Southern | CC | 2 | 2022 | Gas | 700 |
Mawa | Dhaka | CC | 4 | 2023 | Gas | 700 |
Khulna New | Western | CC | 1 | 2023 | Gas | 700 |
Khulna New | Western | CT | 1 | 2023 | Gas | 150 |
Ghorasal | Dhaka | CC | 1 | 2024 | Gas | 700 |
Khulna New | Western | CC | 2 | 2024 | Gas | 700 |
Ashuganj | Dhaka | CC | 1 | 2025 | Gas | 700 |
Fenchuganj | Central | CT | 2 | 2025 | Gas | 150 |
Baghabari | Northern | CT | 2 | 2025 | Gas | 150 |
Rangpur | Northern | CT | 1 | 2025 | Gas | 150 |
Bheramara | Western | CT | 1 | 2025 | Gas | 150 |
Total | | | | | | 20,495 |
6.6.2 High and Low Demand
6.6.2.1 Unit Additions and System Reliability
Tables 6-9 and 6-10 present the unit additions and system reliability measures for the High and Low Demand scenarios. We offer the following observations:
- The additions keep pace with the demand, including a reserve margin.
- Except for the first few years, the system reliability indices are similar in the Base Case and these two scenarios. In the first few years, before significant numbers of new units can be added, the higher the load, the lower the reliability.
- The reserve margins are similar to those in the Base Case. The Low Demand scenario has slightly higher reserve margins, which is to be expected because larger systems need slightly lower reserve margins, all else equal.
- The mixes of new units are similar to that in the Base Case. 150 MW SCGT comprise 19% - 23% in the three scenarios.
Table 6-9 High Demand Scenario Unit Additions and System Reliability Indices
| | Unit Additions, Number of Units | | System Reliabilty Indices |
Year | Peak Load, MW | 700 MW CC | 450 MW CC | 150 MW SCGT | Installed Capacity, MW | LOLP, % | ENS, GWH | Reserve Margin, % |
2005 | 4,381 | 0 | 0 | 0 | 4,458 | 9.278 | 228.6 | 2% |
2006 | 4,839 | 0 | 0 | 0 | 4,683 | 12.976 | 269.3 | -3% |
2007 | 5,345 | 0 | 0 | 0 | 5,425 | 9.341 | 80.5 | 1% |
2008 | 5,904 | 0 | 2 | 0 | 6,602 | 3.278 | 133.3 | 12% |
2009 | 6,567 | 0 | 1 | 0 | 7,913 | 0.883 | 7.2 | 20% |
2010 | 7,355 | 0 | 2 | 2 | 8,886 | 0.709 | 11.4 | 21% |
2011 | 8,237 | 0 | 2 | 0 | 9,786 | 0.998 | 7.0 | 19% |
2012 | 9,288 | 0 | 3 | 0 | 11,099 | 0.805 | 10.2 | 19% |
2013 | 10,473 | 0 | 3 | 0 | 12,379 | 0.983 | 10.7 | 18% |
2014 | 11,810 | 0 | 3 | 2 | 14,029 | 0.771 | 9.8 | 19% |
2015 | 13,408 | 3 | 0 | 0 | 15,779 | 0.983 | 7.9 | 18% |
2016 | 15,223 | 3 | 0 | 0 | 17,879 | 0.982 | 9.1 | 17% |
2017 | 17,166 | 2 | 0 | 6 | 20,179 | 0.844 | 10.0 | 18% |
2018 | 19,357 | 3 | 0 | 2 | 22,579 | 0.965 | 13.5 | 17% |
2019 | 21,827 | 0 | 0 | 19 | 25,293 | 0.866 | 10.7 | 16% |
2020 | 24,445 | 2 | 0 | 10 | 28,193 | 0.876 | 10.7 | 15% |
2021 | 27,377 | 5 | 0 | 1 | 31,505 | 0.918 | 14.4 | 15% |
2022 | 30,661 | 5 | 0 | 4 | 35,276 | 0.922 | 15.1 | 15% |
2023 | 34,103 | 6 | 0 | 0 | 39,267 | 0.905 | 13.0 | 15% |
2024 | 37,931 | 5 | 0 | 6 | 43,470 | 0.987 | 7.7 | 15% |
2025 | 41,899 | 5 | 0 | 7 | 48,020 | 0.956 | 16.0 | 15% |
| Total | 39 | 16 | 59 | 114 | | | |
| Total MW | 27,300 | 7,200 | 8,850 | 43,350 | | | |
| Percent | 63% | 17% | 20% | ^ Total Units and MW Added | |
Table 6-10 Low Demand Scenario Unit Additions and System Reliability Indices
| | Unit Additions, Number of Units | | System Reliabilty Indices |
Year | Peak Load, MW | 700 MW CC | 450 MW CC | 150 MW SCGT | Installed Capacity, MW | LOLP, % | ENS, GWH | Reserve Margin, % |
2005 | 4,308 | 0 | 0 | 0 | 4,458 | 8.138 | 180.7 | 3% |
2006 | 4,627 | 0 | 0 | 0 | 4,683 | 9.913 | 269.3 | 1% |
2007 | 4,970 | 0 | 0 | 0 | 5,425 | 4.621 | 80.5 | 9% |
2008 | 5,339 | 0 | 0 | 0 | 5,702 | 5.970 | 133.3 | 7% |
2009 | 5,734 | 0 | 1 | 0 | 7,013 | 0.693 | 7.2 | 22% |
2010 | 6,160 | 0 | 1 | 1 | 7,386 | 0.988 | 11.4 | 20% |
2011 | 6,569 | 0 | 1 | 1 | 7,986 | 0.653 | 7.0 | 22% |
2012 | 7,007 | 0 | 1 | 0 | 8,399 | 0.873 | 10.2 | 20% |
2013 | 7,473 | 0 | 1 | 1 | 8,929 | 0.891 | 10.7 | 19% |
2014 | 7,970 | 0 | 1 | 1 | 9,529 | 0.818 | 9.8 | 20% |
2015 | 8,501 | 0 | 2 | 1 | 10,229 | 0.673 | 7.9 | 20% |
2016 | 9,066 | 0 | 1 | 1 | 10,829 | 0.761 | 9.1 | 19% |
2017 | 9,670 | 0 | 0 | 4 | 11,429 | 0.841 | 10.0 | 18% |
2018 | 10,313 | 1 | 0 | 0 | 12,129 | 0.961 | 13.5 | 18% |
2019 | 11,000 | 1 | 0 | 2 | 12,993 | 0.785 | 10.7 | 18% |
2020 | 11,732 | 1 | 0 | 1 | 13,843 | 0.783 | 10.7 | 18% |
2021 | 12,424 | 1 | 0 | 2 | 14,505 | 0.942 | 14.4 | 17% |
2022 | 13,157 | 1 | 0 | 3 | 15,326 | 0.956 | 15.1 | 16% |
2023 | 13,934 | 1 | 0 | 3 | 16,267 | 0.859 | 13.0 | 17% |
2024 | 14,756 | 2 | 0 | 0 | 17,470 | 0.574 | 7.7 | 18% |
2025 | 15,626 | 1 | 0 | 0 | 18,170 | 0.932 | 16.0 | 16% |
| Total | 9 | 9 | 21 | 39 | | | |
| Total MW | 6,300 | 4,050 | 3,150 | 13,500 | | | |
| Percent | 47% | 30% | 23% | ^ Total Units and MW Added | |
6.6.2.2 Costs and Fuel Requirements
Costs and fuel requirements for natural gas follow the same trend as demand. Figure 6-10 compares those parameters for the three scenarios. Base Case demand is much closer to Low Demand scenario demand, so Base Case cost and fuel use are much closer to Low Demand scenario values than to High Demand scenario values.
The High and Low Demand scenarios differ little compared to the Base Case in fuel requirements for domestic coal, fuel oil, and diesel. Therefore we do not show detailed data on those fuels.
Figure 6-10 Demand Scenarios - Cost and Fuel Use Comparison
6.6.3 Coal Scenarios
6.6.3.1 Limited Gas
Unit Additions and System Reliability
Table 6-11 presents the unit additions and system reliability measures for the Limited Gas scenario. We offer the following observations:
- Slightly more MW of new units are added than in the Base Case, compensating for the coal plants’ lower reliability.
- The reliability statistics are similar to the Base Case, except for slightly higher reserve margins, again compensating for the coal plants’ lower reliability. There is variation compared to the Base Case from year to year because the coal units are not exactly the same size as the 450 MW and 700 MW combined cycles they replace.
- The peaking SCGT represent 19% of the MW of the new units added, about the same as in the Base Case.
Table 6-11 Limited Gas Scenario Unit Additions and System Reliability Indices
| | Unit Additions, Number of Units | | | | System Reliabilty Indices |
Year | Peak Load, MW | 700 MW CC | 450 MW CC | 150 MW SCGT | 500 MW 500 MW Dom Imp Coal Coal | Installed Capacity, MW | LOLP, % | ENS, GWH | Reserve Margin, % |
2005 | 4,308 | 0 | 0 | 0 | 0 | 0 | 4,458 | 8.138 | 180.7 | 3% |
2006 | 4,693 | 0 | 0 | 0 | 0 | 0 | 4,683 | 10.884 | 320.9 | 0% |
2007 | 5,112 | 0 | 0 | 0 | 0 | 0 | 5,425 | 6.350 | 137.4 | 6% |
2008 | 5,569 | 0 | 0 | 2 | 0 | 0 | 6,002 | 5.135 | 108.4 | 8% |
2009 | 6,066 | 0 | 1 | 0 | 0 | 0 | 7,313 | 0.845 | 8.8 | 21% |
2010 | 6,608 | 0 | 2 | 0 | 0 | 0 | 7,986 | 0.750 | 8.1 | 21% |
2011 | 7,148 | 0 | 1 | 1 | 0 | 0 | 8,586 | 0.797 | 9.1 | 20% |
2012 | 7,732 | 0 | 2 | 0 | 0 | 0 | 9,449 | 0.490 | 5.1 | 22% |
2013 | 8,364 | 0 | 1 | 1 | 0 | 0 | 9,979 | 0.834 | 10.2 | 19% |
2014 | 9,047 | 0 | 2 | 0 | 0 | 0 | 10,879 | 0.654 | 7.4 | 20% |
2015 | 9,786 | 0 | 2 | 1 | 0 | 0 | 11,579 | 0.937 | 12.6 | 18% |
2016 | 10,512 | 0 | 0 | 5 | 0 | 0 | 12,329 | 0.984 | 12.8 | 17% |
2017 | 11,291 | 0 | 0 | 0 | 2 | 0 | 13,329 | 0.954 | 13.0 | 18% |
2018 | 12,128 | 0 | 0 | 6 | 0 | 0 | 14,229 | 0.932 | 11.6 | 17% |
2019 | 13,027 | 0 | 0 | 0 | 3 | 0 | 15,593 | 0.622 | 6.7 | 20% |
2020 | 13,993 | 0 | 0 | 0 | 2 | 0 | 16,593 | 0.890 | 12.3 | 19% |
2021 | 14,924 | 0 | 0 | 3 | 1 | 1 | 17,705 | 0.859 | 11.6 | 19% |
2022 | 15,917 | 0 | 0 | 0 | 0 | 3 | 18,876 | 0.998 | 16.3 | 19% |
2023 | 16,977 | 0 | 0 | 1 | 0 | 3 | 20,317 | 0.837 | 12.1 | 20% |
2024 | 18,107 | 0 | 0 | 0 | 0 | 3 | 21,620 | 0.953 | 16.0 | 19% |
2025 | 19,312 | 0 | 0 | 3 | 0 | 2 | 23,070 | 0.918 | 14.7 | 19% |
| Total | 0 | 11 | 23 | 8 | 12 | 43 | | | |
| Total MW | 0 | 4,950 | 3,450 | 4,000 | 6,000 | 18,400 | | | |
| Percent | 0% | 27% | 19% | 22% | 33% | ^ Total Units and MW Added | |
Costs
Table 6-12 summarizes the costs for the Limited Gas scenario. The discounted total cost of
$14,718 million exceeds the corresponding Base Case value of $13,050 million by $1,668
million. On an undiscounted basis the difference of $8,323 million is much larger because all of the yearly advantages of the Base Case come in 2012 and thereafter and thus have many years of discounting.
The sums of fuel and variable O&M costs for the coal plants are lower than the corresponding sums for the CC plants. However, the coal plants’ capital costs far outweigh the relatively small advantage in operating cost.
These cost results are based on a natural gas price of $3.02/GJ. The advantage of natural gas would increase if lower natural gas prices were used.
Table 6-12 Limited Gas Scenario System Costs
| All Costs in Millions of 2005 US$ |
Year | Operating Costs | Capital Costs | Total Direct Costs | ENS Costs | Total Costs |
Fixed & Variable O&M | Fuel |
2005 | 124 | 702 | 0 | 826 | 78 | 904 |
2006 | 138 | 738 | 63 | 938 | 138 | 1,076 |
2007 | 157 | 811 | 305 | 1,273 | 59 | 1,332 |
2008 | 161 | 867 | 478 | 1,506 | 47 | 1,552 |
2009 | 180 | 924 | 467 | 1,570 | 4 | 1,574 |
2010 | 185 | 964 | 518 | 1,667 | 4 | 1,670 |
2011 | 193 | 1,036 | 467 | 1,696 | 4 | 1,700 |
2012 | 202 | 1,101 | 589 | 1,892 | 2 | 1,894 |
2013 | 210 | 1,177 | 765 | 2,152 | 4 | 2,156 |
2014 | 220 | 1,258 | 847 | 2,325 | 3 | 2,328 |
2015 | 232 | 1,347 | 1,015 | 2,594 | 5 | 2,599 |
2016 | 244 | 1,476 | 1,249 | 2,969 | 6 | 2,975 |
2017 | 258 | 1,542 | 1,663 | 3,463 | 6 | 3,469 |
2018 | 273 | 1,693 | 1,676 | 3,642 | 5 | 3,647 |
2019 | 281 | 1,530 | 1,806 | 3,616 | 3 | 3,619 |
2020 | 297 | 1,614 | 2,101 | 4,012 | 5 | 4,017 |
2021 | 306 | 1,687 | 2,000 | 3,993 | 5 | 3,998 |
2022 | 320 | 1,778 | 1,624 | 3,722 | 7 | 3,729 |
2023 | 337 | 1,878 | 958 | 3,173 | 5 | 3,178 |
2024 | 353 | 1,980 | 394 | 2,726 | 7 | 2,733 |
2025 | 373 | 2,105 | 0 | 2,478 | 6 | 2,484 |
Total | 5,041 | 28,207 | 18,984 | 52,233 | 403 | 52,635 |
Total NPV | 1,485 | 8,202 | 4,760 | 14,447 | 270 | 14,718 |
Fuel Requirements
Table 6-13 presents the fuel requirements for the Limited Gas scenario.
In the Limited Gas Scenario, we assume that 4,000 MW of new power plant capacity is developed using domestic coal. In order to achieve that goal, development beyond that planned for Barapukuria and Phulbari would be needed. The reserves listed in Table 4-7 seem to support domestic coal supplies for 4,000 MW of power plant capacity, or possibly even more, as a reasonable expectation.
Natural gas requirement starts to fall below Base Case requirement in 2017, when the first large coal plant comes on line. The difference grows as the number of coal plants increases. Coal use grows starting in 2017 as the new large coal units become operational. By 2025 the total domestic and imported coal requirement is almost 24,000 thousand tones.
Table 6-13 Limited Gas Scenario Fuel Requirements
| Base Case Gas Use, Billion Cubic Ft | Limited Gas Scenario Fuel Use |
Year | Gas, Billion Cubic Ft | Fuel Use in Thousand Metric Tonnes |
Domestic Coal | Imported Coal | Fuel Oil | HS Diesel |
2005 | 225 | 225 | 0 | 0 | 274 | 42 |
2006 | 231 | 231 | 327 | 0 | 281 | 55 |
2007 | 255 | 255 | 644 | 0 | 262 | 32 |
2008 | 277 | 277 | 635 | 0 | 248 | 5 |
2009 | 304 | 304 | 569 | 0 | 163 | 0 |
2010 | 318 | 318 | 530 | 0 | 161 | 0 |
2011 | 344 | 344 | 523 | 0 | 162 | 0 |
2012 | 367 | 367 | 507 | 0 | 154 | 0 |
2013 | 393 | 393 | 509 | 0 | 161 | 0 |
2014 | 422 | 422 | 500 | 0 | 159 | 0 |
2015 | 457 | 457 | 498 | 0 | 84 | 0 |
2016 | 487 | 502 | 503 | 0 | 83 | 0 |
2017 | 534 | 490 | 2,789 | 0 | 83 | 0 |
2018 | 572 | 542 | 2,800 | 0 | 84 | 0 |
2019 | 543 | 427 | 6,974 | 0 | 0 | 0 |
2020 | 579 | 419 | 9,431 | 0 | 0 | 0 |
2021 | 607 | 403 | 10,729 | 1,184 | 0 | 0 |
2022 | 641 | 376 | 10,688 | 4,551 | 0 | 0 |
2023 | 678 | 358 | 10,656 | 7,645 | 0 | 0 |
2024 | 717 | 340 | 10,715 | 10,632 | 0 | 0 |
2025 | 762 | 343 | 10,839 | 12,853 | 0 | 0 |
Total | 9,713 | 7,790 | 81,365 | 36,865 | 2,357 | 134 |
6.6.3.2 Limited Gas – Early Coal
Unit Additions and System Reliability
Table 6-14 presents the unit additions and system reliability measures for the Limited Gas – Early Coal scenario. We offer the following observations:
- The total MW of new units added is identical to the Limited Gas scenario and slightly higher than in the Base Case, again compensating for the coal plants’ lower reliability.
- The 450 MW combined cycles come in over the period 2009 – 2018 rather than 2009 – 2015. In only one year do two of these combined cycles come on line, compared to four years for the Limited Gas scenario.
- The reliability statistics are similar to the Base Case and the Limited Gas scenario, except for slightly higher reserve margins, again compensating for the coal plants’ lower reliability. There is variation from year to year because the coal units are not exactly the same size as the 450 MW and 700 MW combined cycles they replace or defer.
- The peaking SCGT represent 19% of the MW of the new units added, about the same as in the Base Case.
Table 6-14 Limited Gas – Early Coal Scenario Unit Additions and System Reliability Indices
| | Unit Additions, Number of Units | | | | System Reliabilty Indices |
Year | Peak Load, MW | 700 MW CC | 450 MW CC | 150 MW SCGT | 500 MW 500 MW Dom Imp Coal Coal | Installed Capacity, MW | LOLP, % | ENS, GWH | Reserve Margin, % |
2005 | 4,308 | 0 | 0 | 0 | 0 | 0 | 4,458 | 8.138 | 180.8 | 4% |
2006 | 4,693 | 0 | 0 | 0 | 0 | 0 | 4,683 | 10.884 | 320.9 | 0% |
2007 | 5,112 | 0 | 0 | 0 | 0 | 0 | 5,425 | 6.350 | 137.5 | 6% |
2008 | 5,569 | 0 | 0 | 2 | 0 | 0 | 6,002 | 5.135 | 108.3 | 8% |
2009 | 6,066 | 0 | 1 | 0 | 0 | 0 | 7,313 | 0.845 | 8.9 | 21% |
2010 | 6,608 | 0 | 2 | 0 | 0 | 0 | 7,986 | 0.750 | 8.2 | 21% |
2011 | 7,148 | 0 | 1 | 1 | 0 | 0 | 8,586 | 0.797 | 9.0 | 20% |
2012 | 7,732 | 0 | 1 | 0 | 1 | 0 | 9,499 | 0.471 | 5.0 | 23% |
2013 | 8,364 | 0 | 1 | 1 | 0 | 0 | 10,029 | 0.823 | 10.2 | 20% |
2014 | 9,047 | 0 | 1 | 0 | 1 | 0 | 10,979 | 0.633 | 7.5 | 21% |
2015 | 9,786 | 0 | 1 | 1 | 1 | 0 | 11,729 | 0.885 | 12.6 | 20% |
2016 | 10,512 | 0 | 1 | 2 | 0 | 0 | 12,479 | 0.995 | 14.6 | 19% |
2017 | 11,291 | 0 | 1 | 0 | 1 | 0 | 13,429 | 0.968 | 14.2 | 19% |
2018 | 12,128 | 0 | 1 | 1 | 1 | 0 | 14,529 | 0.790 | 10.6 | 20% |
2019 | 13,027 | 0 | 0 | 4 | 1 | 0 | 15,493 | 0.876 | 12.3 | 19% |
2020 | 13,993 | 0 | 0 | 4 | 1 | 0 | 16,593 | 0.890 | 12.3 | 19% |
2021 | 14,924 | 0 | 0 | 3 | 1 | 1 | 17,705 | 0.859 | 11.5 | 19% |
2022 | 15,917 | 0 | 0 | 0 | 0 | 3 | 18,876 | 0.998 | 16.2 | 19% |
2023 | 16,977 | 0 | 0 | 1 | 0 | 3 | 20,317 | 0.837 | 12.2 | 20% |
2024 | 18,107 | 0 | 0 | 0 | 0 | 3 | 21,620 | 0.953 | 16.0 | 19% |
2025 | 19,312 | 0 | 0 | 3 | 0 | 2 | 23,070 | 0.918 | 14.6 | 20% |
| Total | 0 | 11 | 23 | 8 | 12 | 43 | | | |
| Total MW | 0 | 4,950 | 3,450 | 4,000 | 6,000 | 18,400 | | | |
| Percent | 0% | 27% | 19% | 22% | 33% | ^ Total Units and MW Added | |
Costs
Table 6-15 summarizes the costs for the Limited Gas – Early Coal scenario. The early introduction of coal units reduces fuel costs by $83 million in net present value compared to the Limited Gas scenario. However, it increases O&M by $5 million and capital costs by
$287 million, resulting in a net cost increase of $209 million in net present value. Despite the coal units’ fuel cost advantage, their total costs are higher than the combined cycles they replace. Therefore introducing them earlier increases overall costs on a net present value basis.
Table 6-15 Limited Gas – Early Coal Scenario System Costs
| All Costs in Millions of 2005 US$ |
Year | Operating Costs | Capital Costs | Total Direct Costs | ENS Costs | Total Costs |
Fixed & Variable O&M | Fuel |
2005 | 124 | 702 | 0 | 826 | 78 | 904 |
2006 | 138 | 738 | 63 | 938 | 138 | 1,076 |
2007 | 157 | 811 | 326 | 1,294 | 59 | 1,353 |
2008 | 161 | 867 | 570 | 1,598 | 47 | 1,644 |
2009 | 180 | 924 | 676 | 1,779 | 4 | 1,783 |
2010 | 185 | 964 | 711 | 1,859 | 4 | 1,863 |
2011 | 193 | 1,036 | 770 | 1,999 | 4 | 2,003 |
2012 | 203 | 1,088 | 834 | 2,125 | 2 | 2,127 |
2013 | 211 | 1,164 | 825 | 2,200 | 4 | 2,204 |
2014 | 223 | 1,232 | 849 | 2,304 | 3 | 2,307 |
2015 | 236 | 1,307 | 966 | 2,509 | 5 | 2,515 |
2016 | 247 | 1,410 | 1,033 | 2,691 | 6 | 2,697 |
2017 | 260 | 1,490 | 1,070 | 2,820 | 6 | 2,826 |
2018 | 274 | 1,586 | 1,384 | 3,244 | 5 | 3,249 |
2019 | 281 | 1,517 | 1,830 | 3,628 | 5 | 3,633 |
2020 | 297 | 1,614 | 2,101 | 4,012 | 5 | 4,017 |
2021 | 306 | 1,687 | 2,000 | 3,993 | 5 | 3,998 |
2022 | 320 | 1,778 | 1,624 | 3,722 | 7 | 3,729 |
2023 | 337 | 1,878 | 958 | 3,173 | 5 | 3,178 |
2024 | 353 | 1,980 | 394 | 2,726 | 7 | 2,733 |
2025 | 373 | 2,105 | 0 | 2,478 | 6 | 2,484 |
Total | 5,057 | 27,877 | 18,984 | 51,918 | 406 | 52,323 |
Total NPV | 1,490 | 8,119 | 5,047 | 14,656 | 271 | 14,927 |
Fuel Requirements
Table 6-16 presents the fuel requirements for the Limited Gas – Early Coal scenario. The earlier introduction of coal increases coal use in the early years while reducing gas use compared to the Limited Gas Scenario.
Table 6-16 Limited Gas – Early Coal Scenario Fuel Requirements
Year | Gas, Billion SCF | Fuel Use in Thousand Metric Tonnes |
Domestic Coal | Imported Coal | Fuel Oil | HS Diesel |
2005 | 225 | 0 | 0 | 274 | 42 |
2006 | 231 | 327 | 0 | 281 | 55 |
2007 | 255 | 644 | 0 | 262 | 32 |
2008 | 277 | 635 | 0 | 248 | 5 |
2009 | 304 | 569 | 0 | 163 | 0 |
2010 | 318 | 530 | 0 | 161 | 0 |
2011 | 344 | 523 | 0 | 162 | 0 |
2012 | 346 | 1,606 | 0 | 152 | 0 |
2013 | 371 | 1,654 | 0 | 160 | 0 |
2014 | 379 | 2,704 | 0 | 159 | 0 |
2015 | 393 | 3,780 | 0 | 82 | 0 |
2016 | 428 | 3,835 | 0 | 83 | 0 |
2017 | 440 | 4,863 | 0 | 84 | 0 |
2018 | 459 | 5,834 | 0 | 82 | 0 |
2019 | 405 | 8,095 | 0 | 0 | 0 |
2020 | 419 | 9,431 | 0 | 0 | 0 |
2020 | 403 | 10,729 | 1,184 | 0 | 0 |
2020 | 376 | 10,688 | 4,551 | 0 | 0 |
2023 | 358 | 10,656 | 7,645 | 0 | 0 |
2024 | 340 | 10,715 | 10,632 | 0 | 0 |
2025 | 343 | 10,839 | 12,853 | 0 | 0 |
Total | 7,412 | 98,657 | 36,865 | 2,352 | 134 |
6.6.3.3 Fuel Security
Unit Additions and System Reliability
Table 6-17 presents the unit additions and system reliability measures for the Fuel Security scenario. We offer the following observations:
- The total MW of new units added is slightly lower than the Limited Gas – Early Coal scenario and slightly higher than in the Base Case, again compensating for the coal plants’ lower reliability.
- The 450 MW combined cycles come in over the period 2009 – 2018 rather than 2009 – 2015. In only one year do two of these combined cycles come on line, compared to four years for the Limited Gas scenario.
- The reliability statistics are similar to the Base Case and the Limited Gas scenario, except for slightly higher reserve margins than the Base Case, again compensating for the coal plants’ lower reliability. There is variation from year to year because the coal units are not exactly the same size as the 450 MW and 700 MW combined cycles they replace or defer.
- The peaking SCGT represent 19% of the MW of the new units added, about the same as in the Base Case.
Table 6-17 Fuel Security Scenario Unit Additions and System Reliability Indices
| | Unit Additions, Number of Units | | System Reliabilty Indices |
Year | Peak Load, MW | 700 MW CC | 450 MW CC | 150 MW SCGT | 500 MW Dom Coal | 500 MW Imp Coal | Installed Capacity, MW | LOLP, % | ENS, GWH | Reserve Margin, % |
2005 | 4,308 | 0 | 0 | 0 | 0 | 0 | 4,458 | 8.138 | 180.8 | 4% |
2006 | 4,693 | 0 | 0 | 0 | 0 | 0 | 4,683 | 10.884 | 320.9 | 0% |
2007 | 5,112 | 0 | 0 | 0 | 0 | 0 | 5,425 | 6.350 | 137.5 | 6% |
2008 | 5,569 | 0 | 0 | 2 | 0 | 0 | 6,002 | 5.135 | 108.3 | 8% |
2009 | 6,066 | 0 | 1 | 0 | 0 | 0 | 7,313 | 0.845 | 8.9 | 21% |
2010 | 6,608 | 0 | 2 | 0 | 0 | 0 | 7,986 | 0.750 | 8.2 | 21% |
2011 | 7,148 | 0 | 1 | 1 | 0 | 0 | 8,586 | 0.797 | 9.0 | 20% |
2012 | 7,732 | 0 | 1 | 0 | 1 | 0 | 9,499 | 0.471 | 5.0 | 23% |
2013 | 8,364 | 0 | 1 | 1 | 0 | 0 | 10,029 | 0.823 | 10.2 | 20% |
2014 | 9,047 | 0 | 1 | 0 | 1 | 0 | 10,979 | 0.633 | 7.5 | 21% |
2015 | 9,786 | 0 | 1 | 1 | 1 | 0 | 11,729 | 0.885 | 12.6 | 20% |
2016 | 10,512 | 0 | 1 | 2 | 0 | 0 | 12,479 | 0.995 | 14.6 | 19% |
2017 | 11,291 | 0 | 1 | 3 | 0 | 0 | 13,429 | 0.968 | 14.2 | 19% |
2018 | 12,128 | 1 | 0 | 2 | 0 | 0 | 14,529 | 0.790 | 10.6 | 20% |
2019 | 13,027 | 0 | 0 | 4 | 1 | 0 | 15,493 | 0.876 | 12.3 | 19% |
2020 | 13,993 | 1 | 0 | 0 | 1 | 0 | 16,593 | 0.890 | 12.3 | 19% |
2021 | 14,924 | 1 | 0 | 1 | 1 | 0 | 17,705 | 0.859 | 11.5 | 19% |
2022 | 15,917 | 1 | 0 | 2 | 1 | 0 | 18,876 | 0.998 | 16.2 | 19% |
2023 | 16,977 | 1 | 1 | 0 | 1 | 0 | 20,317 | 0.837 | 12.2 | 20% |
2024 | 18,107 | 2 | 0 | 0 | 0 | 0 | 21,620 | 0.953 | 16.0 | 19% |
2025 | 19,312 | 1 | 0 | 4 | 0 | 0 | 23,070 | 0.918 | 14.6 | 20% |
| Total | 8 | 11 | 23 | 8 | 0 | 31 | | | |
| Total MW | 5,600 | 4,950 | 3,450 | 4,000 | 0 | 18,000 | | | |
| Percent | 31% | 28% | 19% | 22% | 0% | ^ Total Units and MW Added | |
Costs
Table 6-18 summarizes the costs for the Fuel Security scenario. This scenario is closer to the Base Case in coal plant additions, so is closer in costs as well.
Table 6-18 Fuel Security Scenario System Costs
| All Costs in Millions of 2005 US$ |
Year | Operating Costs | Capital Costs | Total Direct Costs | ENS Costs | Total Costs |
Fixed & Variable O&M | Fuel |
2005 | 124 | 702 | 0 | 826 | 78 | 904 |
2006 | 138 | 738 | 63 | 938 | 138 | 1,076 |
2007 | 157 | 811 | 326 | 1,294 | 59 | 1,353 |
2008 | 161 | 867 | 570 | 1,598 | 47 | 1,644 |
2009 | 180 | 924 | 676 | 1,779 | 4 | 1,783 |
2010 | 185 | 964 | 711 | 1,859 | 4 | 1,863 |
2011 | 193 | 1,036 | 770 | 1,999 | 4 | 2,003 |
2012 | 203 | 1,088 | 813 | 2,103 | 2 | 2,105 |
2013 | 211 | 1,164 | 712 | 2,087 | 4 | 2,091 |
2014 | 223 | 1,232 | 543 | 1,997 | 3 | 2,001 |
2015 | 236 | 1,307 | 564 | 2,107 | 5 | 2,113 |
2016 | 247 | 1,410 | 818 | 2,476 | 6 | 2,482 |
2017 | 260 | 1,529 | 911 | 2,700 | 5 | 2,705 |
2018 | 273 | 1,640 | 1,132 | 3,045 | 5 | 3,050 |
2019 | 278 | 1,536 | 1,116 | 2,930 | 6 | 2,936 |
2020 | 294 | 1,627 | 1,110 | 3,031 | 6 | 3,037 |
2021 | 302 | 1,699 | 1,078 | 3,079 | 7 | 3,086 |
2022 | 313 | 1,788 | 879 | 2,981 | 7 | 2,988 |
2023 | 327 | 1,890 | 589 | 2,806 | 5 | 2,811 |
2024 | 339 | 1,997 | 309 | 2,646 | 6 | 2,652 |
2025 | 357 | 2,126 | 0 | 2,483 | 7 | 2,490 |
Total | 5,000 | 28,073 | 13,690 | 46,764 | 408 | 47,171 |
Total NPV | 1,483 | 8,152 | 4,065 | 13,701 | 271 | 13,972 |
Fuel Requirements
Table 6-19 presents the fuel requirements for the Fuel Security scenario. Fuel use shows natural gas use and coal use intermediate between the Base Case and Limited Gas scenario.
Table 6-19 Fuel Security Scenario Fuel Requirements
Year | Gas, BCF | Fuel Use in Thousand Metric Tonnes |
Domestic Coal | Imported Coal | Fuel Oil | HS Diesel |
2005 | 225 | 0 | 0 | 274 | 42 |
2006 | 231 | 327 | 0 | 281 | 55 |
2007 | 255 | 644 | 0 | 262 | 32 |
2008 | 277 | 635 | 0 | 248 | 5 |
2009 | 304 | 569 | 0 | 163 | 0 |
2010 | 318 | 530 | 0 | 161 | 0 |
2011 | 344 | 523 | 0 | 162 | 0 |
2012 | 346 | 1,606 | 0 | 152 | 0 |
2013 | 371 | 1,654 | 0 | 160 | 0 |
2014 | 379 | 2,704 | 0 | 159 | 0 |
2015 | 393 | 3,780 | 0 | 82 | 0 |
2016 | 428 | 3,835 | 0 | 83 | 0 |
2017 | 469 | 3,851 | 0 | 82 | 0 |
2018 | 508 | 3,853 | 0 | 83 | 0 |
2019 | 447 | 5,740 | 0 | 0 | 0 |
2020 | 461 | 6,965 | 0 | 0 | 0 |
2020 | 467 | 8,225 | 0 | 0 | 0 |
2020 | 479 | 9,463 | 0 | 0 | 0 |
2023 | 496 | 10,603 | 0 | 0 | 0 |
2024 | 533 | 10,685 | 0 | 0 | 0 |
2025 | 575 | 10,839 | 0 | 0 | 0 |
Total | 8,304 | 87,032 | 0 | 2,352 | 134 |
6.6.3.4 Comparison of Coal Scenarios and Base Case
Unit Additions and System Reliability
The reliability statistics are similar among the four scenarios, with some variation from year to year. The reserve margins increase slightly as the total capacity of installed coal plants increases. Total installed capacity also increases as the fraction devoted to coal plants increases because they are less reliable and have longer maintenance outages than the combined cycles they replace. Table 6-20 summarizes the unit additions in the four cases.
Table 6-20 Unit Additions in Coal Scenarios and Base Case
Unit Type | Total Capacity Additions, MW |
Base Case | Limited Gas | Limited Gas - Early Coal | Fuel Security |
750 MW Combined Cycle | 8,400 | 0 | 0 | 5,600 |
450 MW Combined Cycle | 5,850 | 4,950 | 4,950 | 4,950 |
150 MW Simple Cycle Gas Turbine | 3,450 | 3,450 | 3,450 | 3,450 |
500 MW Domestic Coal Units | 0 | 4,000 | 4,000 | 4,000 |
500 MW Imported Coal Units | 0 | 6,000 | 6,000 | 0 |
TOTAL | 17,700 | 18,400 | 18,400 | 18,000 |
MW Above Base Case Total | 0 | 700 | 700 | 300 |
Costs
Figure 6-11 compares the yearly total costs for the four scenarios. The Base Case has the lowest costs. For the coal scenarios, in general more coal plants means higher costs, and earlier introduction means higher costs. The relationships varies among the years depending on which units are under construction and how many have already been built. Table 6-21 summarizes the study period costs for the four scenarios.
Figure 6-11 Total Costs in Coal Scenarios and Base Case
Table 6-21 Total Costs by Cost Category in Coal Scenarios and Base Case
Cost Category | Study Period Costs, $ Millions |
Base Case | Base Case | Lmtd Gas | Lmtd Gas | Lmtd Gas - Early Coal | Lmtd Gas - Early Coal | Fuel Secur- ity | Fuel Secur- ity |
Total | NPV | Total | NPV | Total | NPV | Total | NPV |
Operating: | | | | | | | | |
O&M | 4,916 | 1,469 | 5,041 | 1,485 | 5,057 | 1,490 | 5,000 | 1,483 |
Fuel | 28,758 | 8,268 | 28,207 | 8,202 | 27,877 | 8,119 | 28,073 | 8,152 |
Total Operating | | | | | | | | |
Capital | 10,236 | 3,042 | 18,984 | 4,760 | 18,984 | 5,047 | 13,690 | 4,065 |
Total Direct | 43,910 | 12,779 | 52,233 | 14,447 | 51,918 | 14,656 | 46,764 | 13,701 |
Cost of Energy Not Served | 402 | 270 | 403 | 270 | 406 | 271 | 408 | 271 |
Total Cost | 44,313 | 13,050 | 52,635 | 14,718 | 52,323 | 14,927 | 47,171 | 13,972 |
Fuel Requirements
Figure 6-12 shows the natural gas and coal fuel requirements for the four scenarios. All the coal scenarios use substantially less natural gas compared to the Base Case by the end of the study period, and far more coal.
The Limited Gas and Limited Gas – Early Coal scenarios have similar impact on natural gas use and similar use of coal, except during the early years when the installation of coal plants is advanced in the Early Coal scenario. By the end of the study period in 2025, annual natural gas use is less than half of the Base Case in both those scenarios. Naturally, the Fuel Security scenario, with fewer MW of coal plants, reduces natural gas use less and uses less coal than the other two coal scenarios. By 2025 natural gas use is about 75% of that in the Base Case.
Table 6-22 summarizes fuel use over the study period for the four scenarios. The impact on natural gas use over the study period is less dramatic than the impact in the year 2025. The buildup of coal plant capacity from 2012 on provides more impact on gas use in the out years in all the coal scenarios.
Table 6-22 Study Period Natural Gas and Coal Use in Coal Scenarios and Base Case
Scenario | Fuel Use Over Study Period 2005 - 2025 |
Natural Gas, Billion SCF | Coal Use, Thousand Metric Tonnes |
Base Case | 9,713 | 10,297 |
Limited Gas | 7,790 | 118,230 |
Limited Gas - Early Coal | 7,412 | 135,522 |
Fuel Security | 8,304 | 87,032 |
Figure 6-12 Natural Gas and Coal Use in Coal Scenarios and Base Case
6.6.4 High and Low Discount Rate
6.6.4.1 Unit Additions and System Reliability
Tables 6-23 and 6-24 present the unit additions and system reliability measures for the High and Low Discount Rate scenarios. The unit additions are exactly the same in the High Discount Rate scenario as in the Base Case. The Low Discount Rate scenario varies only slightly from the other two. Figure 6-13 plots the total installed capacity.
Figure 6-13 Discount Rate Scenarios - Total Installed Capacity
In the years not shown the total installed capacity is the same in all three scenarios.
Table 6-23 High Disc Rate Scenario Unit Additions and System Reliability Indices
| | Unit Additions, Number of Units | | System Reliabilty Indices |
Year | Peak Load, MW | 700 MW CC | 450 MW CC | 150 MW SCGT | Installed Capacity, MW | LOLP, % | ENS, GWH | Reserve Margin, % |
2005 | 4,308 | 0 | 0 | 0 | 4,458 | 8.138 | 180.7 | 3% |
2006 | 4,693 | 0 | 0 | 0 | 4,683 | 10.884 | 320.9 | 0% |
2007 | 5,112 | 0 | 0 | 0 | 5,425 | 6.350 | 137.4 | 6% |
2008 | 5,569 | 0 | 0 | 2 | 6,002 | 5.135 | 108.4 | 8% |
2009 | 6,066 | 0 | 1 | 0 | 7,313 | 0.845 | 8.8 | 21% |
2010 | 6,608 | 0 | 2 | 0 | 7,986 | 0.750 | 8.1 | 21% |
2011 | 7,148 | 0 | 1 | 1 | 8,586 | 0.797 | 9.1 | 20% |
2012 | 7,732 | 0 | 2 | 0 | 9,449 | 0.490 | 5.1 | 22% |
2013 | 8,364 | 0 | 1 | 1 | 9,979 | 0.834 | 10.2 | 19% |
2014 | 9,047 | 0 | 2 | 0 | 10,879 | 0.654 | 7.4 | 20% |
2015 | 9,786 | 0 | 2 | 1 | 11,579 | 0.937 | 12.6 | 18% |
2016 | 10,512 | 0 | 2 | 0 | 12,479 | 0.848 | 11.2 | 19% |
2017 | 11,291 | 0 | 0 | 5 | 13,229 | 0.997 | 13.5 | 17% |
2018 | 12,128 | 1 | 0 | 2 | 14,229 | 0.912 | 12.3 | 17% |
2019 | 13,027 | 1 | 0 | 3 | 15,243 | 0.880 | 11.9 | 17% |
2020 | 13,993 | 2 | 0 | 0 | 16,643 | 0.578 | 6.7 | 19% |
2021 | 14,924 | 1 | 0 | 3 | 17,455 | 0.816 | 11.2 | 17% |
2022 | 15,917 | 2 | 0 | 0 | 18,526 | 0.949 | 15.6 | 16% |
2023 | 16,977 | 2 | 0 | 1 | 19,867 | 0.811 | 12.6 | 17% |
2024 | 18,107 | 2 | 0 | 0 | 21,070 | 0.923 | 15.8 | 16% |
2025 | 19,312 | 1 | 0 | 4 | 22,370 | 0.950 | 16.0 | 16% |
| Total | 12 | 13 | 23 | 23 | | | |
| Total MW | 8,400 | 5,850 | 3,450 | 17,700 | | | |
| Percent | 47% | 33% | 19% | ^ Total Units and MW Added | |
As one would expect, the reliability statistics are nearly identical in the three scenarios.
A lower discount rate makes long-term savings relatively more important, favoring CC units compared to SCGT units. Even though the total number of each type of unit added is the same in all three scenarios, in the Low Discount Rate scenario some combined cycles are added earlier and some gas turbines later than in the other scenarios.
A high discount rate has the opposite impact, but the results show that the impact was not significant enough to change the resource additions at all compared to the Base Case.
Table 6-24 Low Disc Rate Scenario Unit Additions and System Reliability Indices
| | Unit Additions, Number of Units | | System Reliabilty Indices |
Year | Peak Load, MW | 700 MW CC | 450 MW CC | 150 MW SCGT | Installed Capacity, MW | LOLP, % | ENS, GWH | Reserve Margin, % |
2005 | 4,308 | 0 | 0 | 0 | 4,458 | 8.138 | 180.8 | 3% |
2006 | 4,693 | 0 | 0 | 0 | 4,683 | 10.884 | 320.9 | 0% |
2007 | 5,112 | 0 | 0 | 0 | 5,425 | 6.350 | 137.5 | 6% |
2008 | 5,569 | 0 | 0 | 2 | 6,002 | 5.135 | 108.3 | 8% |
2009 | 6,066 | 0 | 1 | 0 | 7,313 | 0.845 | 8.9 | 21% |
2010 | 6,608 | 0 | 2 | 0 | 7,986 | 0.750 | 8.2 | 21% |
2011 | 7,148 | 0 | 2 | 0 | 8,886 | 0.345 | 3.2 | 24% |
2012 | 7,732 | 0 | 1 | 0 | 9,299 | 0.767 | 9.2 | 20% |
2013 | 8,364 | 0 | 2 | 0 | 10,129 | 0.617 | 7.1 | 21% |
2014 | 9,047 | 0 | 1 | 1 | 10,729 | 0.962 | 12.7 | 19% |
2015 | 9,786 | 0 | 2 | 2 | 11,579 | 0.937 | 12.6 | 18% |
2016 | 10,512 | 0 | 2 | 0 | 12,479 | 0.848 | 11.2 | 19% |
2017 | 11,291 | 0 | 0 | 5 | 13,229 | 0.997 | 13.5 | 17% |
2018 | 12,128 | 2 | 0 | 0 | 14,629 | 0.451 | 5.1 | 21% |
2019 | 13,027 | 1 | 0 | 1 | 15,343 | 0.823 | 11.7 | 18% |
2020 | 13,993 | 1 | 0 | 2 | 16,343 | 0.956 | 14.7 | 17% |
2021 | 14,924 | 1 | 0 | 5 | 17,455 | 0.816 | 11.2 | 17% |
2022 | 15,917 | 2 | 0 | 0 | 18,526 | 0.949 | 15.6 | 16% |
2023 | 16,977 | 2 | 0 | 1 | 19,867 | 0.811 | 12.5 | 17% |
2024 | 18,107 | 2 | 0 | 0 | 21,070 | 0.923 | 15.9 | 16% |
2025 | 19,312 | 1 | 0 | 4 | 22,370 | 0.950 | 16.1 | 16% |
| Total | 12 | 13 | 23 | 23 | | | |
| Total MW | 8,400 | 5,850 | 3,450 | 17,700 | | | |
| Percent | 47% | 33% | 19% | ^ Total Units and MW Added | |
6.6.4.2 Costs
The similarity in the resource additions means that the undiscounted costs are similar. Table 6-25 compares the costs in the three scenarios. The fuel, O&M, and ENS costs are nearly identical. The capital costs differ a little more because the discount rate affects the amount of interest during construction that is included in the capital cost values.
Table 6-25 Discount Rate Scenarios Cost Comparison
| All Costs in Millions of 2005 US$ |
Year | Operating Costs | Capital Costs | Total Direct Costs | ENS Costs | Total Costs |
Fixed & Variable O&M | Fuel |
Base Case | | | | | | |
Total Undiscounted | 4,916 | 28,758 | 10,236 | 43,910 | 402 | 44,313 |
Total NPV | 1,469 | 8,268 | 3,042 | 12,779 | 270 | 13,050 |
High Discount Rate Scenario | | | | | |
Total Undiscounted | 4,916 | 28,758 | 10,588 | 44,261 | 402 | 44,664 |
Total NPV | 1,180 | 6,589 | 2,475 | 10,244 | 252 | 10,496 |
Low Discount Rate Scenario | | | | | |
Total Undiscounted | 4,914 | 28,684 | 10,005 | 43,604 | 403 | 44,006 |
Total NPV | 1,728 | 9,753 | 3,560 | 15,041 | 284 | 15,326 |
6.6.4.3 Fuel Requirements
With nearly identical resource plans, the fuel requirements for the High and Low Discount Rate scenarios are nearly identical to the Base Case requirements and are not shown here.
6.6.5 High and Low Cost of ENS
6.6.5.1 Unit Additions and System Reliability
Figure 6-14 compares total installed capacity in the three scenarios. The Low Cost of ENS scenario has exactly the same resource additions as the Base Case. In the High Cost of ENS scenario, a few unit additions come earlier, but the total installed capacity from 2019 on is the same in all three scenarios.
Figure 6-14 Cost of ENS Scenarios - Total Installed Capacity
A higher cost of ENS rate makes reliability more valuable, favoring the addition of more resources. This resulted in more units in a few years, as shown in Figure 6-14. One 150 MW SCGT comes on line in 2008 instead of 2011. One 700 MW comes on line in 2018 instead of 2019, and two 150 MW SCGT come on line in 2019 rather than 2018. The net impact is 400 MW more in 2018. However, the total resource additions and mix of units is exactly the same in 2019 and thereafter.
A lower cost of ENS makes reliability less valuable, favoring the addition of fewer resources. However, the results show that there is no change to the resource additions compared to the Base Case. An examination of the reliability statistics explains why. The answer is that the LOLP criterion requires more resources than are required by the economic test.
Figure 6-15 presents the LOLP in % for the three scenarios. The criterion of 1% applies from 2009 onward. Only the economic test applies in 2008, and there is not enough time to build new units before then. With more valuable reliability, in the High Cost of ENS scenario more units appear due to the economic test in several years to reduce the LOLP below a level close to the 1% criterion. The results are identical in all three scenarios from 2005 – 2007 and 2019 – 2025.
Figure 6-15 Cost of ENS Scenarios - LOLP
The LOLP criterion produces a resource plan with close to 1% LOLP. It is permissible to add more units in the High scenario to improve reliability. However, with reliability less valuable in the Low scenario, having fewer units would drive the LOLP above 1%, which is not permitted.
6.6.5.2 Costs
The similarity in the resource additions means that all costs except cost of ENS are similar. Table 6-26 compares the costs in the three scenarios. The fuel, O&M, and capital costs are nearly identical. The ENS costs differ in proportion to the unit cost of ENS, but are relatively small compared to the other categories. The rightmost column shows what the total costs are when a unit cost of ENS of $0.43 is applied to each scenario’s ENS in GWH instead of the scenario values for unit cost of ENS. This reduces to almost nothing the cost differences among the scenarios.
Table 6-26 Cost of ENS Scenarios Cost Comparison
| All Costs in Millions of 2005 US$ | |
| Operating Costs | Capital Costs | Total Direct Costs | ENS Costs | Total Costs | Total Costs at Unit Cost of ENS = $0.43/KWH |
Fixed & Variable O&M | Fuel |
Base Case | | | | | | | |
Total Undiscounted | 4,916 | 28,758 | 10,236 | 43,910 | 402 | 44,313 | 44,313 |
Total NPV | 1,469 | 8,268 | 3,042 | 12,779 | 270 | 13,050 | 13,050 |
High Cost of ENS Scenario | | | | | | |
Total Undiscounted | 4,921 | 28,757 | 10,236 | 43,914 | 874 | 44,788 | 44,290 |
Total NPV | 1,472 | 8,276 | 3,062 | 12,810 | 593 | 13,403 | 12,853 |
Low Cost of ENS Scenario | | | | | | |
Total Undiscounted | 4,916 | 28,758 | 10,236 | 43,910 | 187 | 44,097 | 44,312 |
Total NPV | 1,469 | 8,268 | 3,062 | 12,799 | 126 | 12,925 | 13,070 |
6.6.5.3 Fuel Requirements
With nearly identical resource plans, the fuel requirements for the High and Low Cost of ENS scenarios are nearly identical to the Base Case requirements and are not shown here.
6.6.6 No Application of LOLP Criterion
The results of the preceding section raise the question of the impact of the LOLP criterion on overall economics. In this section we remove the LOLP criterion to investigate that point.
We consider the same three values of cost of ENS without that criterion applied.
6.6.6.1 Unit Additions and System Reliability
Figure 6-16 shows the installed capacity and reserve margins for the three scenarios. Both parameters follow the expected pattern. Without the application of an LOLP criterion, lower unit cost of ENS makes reliability less valuable and leads to less capacity and lower reserve margins. The converse is true for higher unit cost of ENS.
Figure 6-17 presents the LOLP and ENS statistics for the three scenarios. As with Figure 6- 16, both the parameters follow the expected pattern. Lower cost of ENS leads to higher LOLP and higher amounts of ENS. The converse is true for higher unit cost of ENS. None of the scenarios achieves LOLP below 1% for all years after 2008. The high cost of ENS scenario has LOLP between 1% and 2% most years after 2008, but never below 1%.
This indicates that application of the LOLP criterion leads to higher overall system costs, given the values for the other parameters we are using. Section 8 discusses this in more detail.
Figure 6-16 No LOLP Scenarios - Total Installed Capacity and Reserve Margins
Figure 6-17 No LOLP Scenarios – Reliability Statistics
6.6.6.2 Costs
The resource additions differ by 1,500 MW, so one would expect substantial differences in costs. Table 6-27 compares the costs in the three scenarios. The main difference is in the capital costs. The ENS costs differ in proportion to the unit cost of ENS. The rightmost column shows what the total costs are when a unit cost of ENS of $0.43 is applied to each scenario’s ENS in GWH instead of the scenario values for unit cost of ENS. This reduces the differences among the economically derived scenarios where no LOLP criterion is applied.
Those three scenarios all offer noticeable benefits compared to the Base Case.
Table 6-27 No LOLP Criterion Scenarios Cost Comparison
| All Costs in Millions of 2005 US$ |
| Operating Costs | Capital Costs | Total Direct Costs | ENS Costs | Total Costs | Total Costs at Unit Cost of ENS = $0.43/KWH |
Fixed & Variable O&M | Fuel |
LOLP Criterion Applied, Base Case Unit Cost of ENS | | | | |
Total Undiscounted | 4,916 | 28,758 | 10,236 | 43,910 | 402 | 44,313 | 44,313 |
Total NPV | 1,469 | 8,268 | 3,042 | 12,779 | 270 | 13,050 | 13,050 |
No LOLP, Base Case Unit Cost of ENS | | | | | |
Total Undiscounted | 4,859 | 28,611 | 9,480 | 42,951 | 928 | 43,879 | 43,879 |
Total NPV | 1,454 | 8,234 | 2,668 | 12,356 | 404 | 12,761 | 12,761 |
No LOLP, High Unit Cost of ENS | | | | | | |
Total Undiscounted | 4,906 | 28,851 | 9,550 | 43,307 | 1,143 | 44,449 | 43,798 |
Total NPV | 1,467 | 8,309 | 2,786 | 12,561 | 655 | 13,217 | 12,843 |
No LOLP, Low Unit Cost of ENS | | | | | | |
Total Undiscounted | 4,821 | 28,583 | 8,838 | 42,241 | 779 | 43,020 | 43,916 |
Total NPV | 1,447 | 8,227 | 2,490 | 12,164 | 259 | 12,423 | 12,721 |
6.6.6.3 Fuel Requirements
As the fuel cost data in Table 6-27 suggests, there is almost no difference among the scenarios in fuel use, so we do not present detailed fuel requirements data here. The incremental capacity above the lowest amount, in the No LOLP - Low Unit Cost of ENS scenario, serves primarily to increase reliability. Not much additional fuel is used because the amount of ENS in any of the scenarios is far less than 1% of energy demand. Thus the maximum potential fuel savings are very small.
6.6.7 Value Scenario – New 500 MW Generating Unit
6.6.7.1 Unit Additions and System Reliability
To summarize, in this analysis we include in the resource plan a 500 MW unit that becomes operational in 2012. Otherwise the scenario is similar to the Base Case. The unit has zero capital, fuel, and O&M costs. It has operating characteristics similar to a coal unit. We extend the period of analysis until 2041 to provide a 30 year life by repeating the 2025 results every year from 2026-2041.
Compared to the Base Case, this “free” plant will reduce the need for other new units and will reduce capital, fuel, and O&M costs. Specifically, one 450 MW CC unit otherwise needed in 2012 is not added to the resource plan. The amount of the overall cost reduction is the potential value to the system of the option of interest.
6.6.7.2 Costs
Table 6-28 presents the cost savings resulting when the costs of this scenario are subtracted from the Base Case values. The 2025 values are extended to 2041 for consistency with an assumed life of 30 years for the new generating plant.
The new 500 MW unit reduces costs equivalent to the unit values summarized in Table 6-29. The values shown are nearly identical to corresponding costs from the screening analysis for a 450 MW CC operating at 75% capacity factor. The Capacity Only Value equals the undiscounted capital costs (corresponding the capital costs at operation) divided by 500 MW. Relatively small differences arise due to differences in reliability and treatment of IDC.
Table 6-28 New 500 MW Unit - Cost Savings
| All Costs in Millions of 2005 US$ | New Unit Parameters |
Year | Operating Costs | Cap- ital Costs | Total Direct Costs | ENS Costs | Total Costs | Genera- tion, GWH | Oper Value, $/MWH | Equiv Cap Fac, % |
Fixed & Var O&M | Fuel |
2005 | 0 | 0 | 0 | 0 | 0 | 0 | 0 | 0 | 0% |
2006 | 0 | 0 | 0 | 0 | 0 | 0 | 0 | 0 | 0% |
2007 | 0 | 0 | 0 | 0 | 0 | 0 | 0 | 0 | 0% |
2008 | 0 | 0 | 0 | 0 | 0 | 0 | 0 | 0 | 0% |
2009 | 0 | 0 | 28 | 28 | 0 | 28 | 0 | 0 | 0% |
2010 | 0 | 0 | 169 | 169 | 0 | 169 | 0 | 0 | 0% |
2011 | 0 | 0 | 113 | 113 | 0 | 113 | 0 | 0 | 0% |
2012 | 7 | 62 | 0 | 69 | 0 | 69 | 2,772 | 25 | 63% |
2013 | 7 | 64 | 0 | 71 | 0 | 71 | 2,898 | 24 | 66% |
2014 | 7 | 63 | 0 | 71 | 0 | 71 | 2,880 | 25 | 66% |
2015 | 7 | 64 | 0 | 72 | 0 | 72 | 2,936 | 24 | 67% |
2016 | 7 | 64 | 0 | 71 | 0 | 72 | 2,925 | 24 | 67% |
2017 | 7 | 65 | 0 | 72 | 0 | 72 | 2,960 | 24 | 68% |
2018 | 7 | 65 | 0 | 72 | 0 | 72 | 2,961 | 24 | 68% |
2019 | 8 | 71 | 0 | 79 | 0 | 79 | 3,340 | 24 | 76% |
2020 | 8 | 70 | 0 | 79 | 0 | 79 | 3,317 | 24 | 76% |
2021 | 8 | 71 | 0 | 79 | 0 | 79 | 3,339 | 24 | 76% |
2022 | 8 | 70 | 0 | 78 | 0 | 78 | 3,331 | 23 | 76% |
2023 | 8 | 70 | 0 | 78 | 0 | 78 | 3,343 | 23 | 76% |
2024 | 8 | 71 | 0 | 79 | 0 | 79 | 3,365 | 24 | 77% |
2025 | 8 | 71 | 0 | 79 | -1 | 78 | 3,365 | 23 | 77% |
2026 | 8 | 71 | 0 | 79 | -1 | 78 | 3,365 | 23 | 77% |
2027 | 8 | 71 | 0 | 79 | -1 | 78 | 3,365 | 23 | 77% |
2028 | 8 | 71 | 0 | 79 | -1 | 78 | 3,365 | 23 | 77% |
2029 | 8 | 71 | 0 | 79 | -1 | 78 | 3,365 | 23 | 77% |
2030 | 8 | 71 | 0 | 79 | -1 | 78 | 3,365 | 23 | 77% |
2031 | 8 | 71 | 0 | 79 | -1 | 78 | 3,365 | 23 | 77% |
2032 | 8 | 71 | 0 | 79 | -1 | 78 | 3,365 | 23 | 77% |
2033 | 8 | 71 | 0 | 79 | -1 | 78 | 3,365 | 23 | 77% |
2034 | 8 | 71 | 0 | 79 | -1 | 78 | 3,365 | 23 | 77% |
2035 | 8 | 71 | 0 | 79 | -1 | 78 | 3,365 | 23 | 77% |
2036 | 8 | 71 | 0 | 79 | -1 | 78 | 3,365 | 23 | 77% |
2037 | 8 | 71 | 0 | 79 | -1 | 78 | 3,365 | 23 | 77% |
2038 | 8 | 71 | 0 | 79 | -1 | 78 | 3,365 | 23 | 77% |
2039 | 8 | 71 | 0 | 79 | -1 | 78 | 3,365 | 23 | 77% |
2040 | 8 | 71 | 0 | 79 | -1 | 78 | 3,365 | 23 | 77% |
2041 | 8 | 71 | 0 | 79 | -1 | 78 | 3,365 | 23 | 77% |
Total | 237 | 2,074 | 309 | 2,620 | -8 | 2,613 | 97,572 | | |
Total NPV | 28 | 243 | 152 | 423 | 0 | 423 | 11,262 | | |
Table 6-29 New 500 MW Unit - Value Summary
| Value of New 500 MW Unit |
| Operating | Total | Capacity Only |
Energy, $/MWH | 24 | 38 | 14 |
Capacity, $/KW | | | 619 |
These results illustrate how much a utility could afford to pay for purchases from a 500 MW unit with the assumed operating characteristics. The Total value is the key result. Any combination of capacity and energy costs that produced the same result in $/MWH at about 75% capacity factor would represent equivalent value.
6.6.8 Value Scenario – New 1,000 MW Interconnection
6.6.8.1 Unit Additions and System Reliability
To summarize, in this analysis we include in the resource plan one 500 MW “free” unit with zero cost fuel that dispatches at nearly 100% capacity factor. At the same time we add another 500 MW plant with such high fuel costs and heat rate that it dispatches at nearly zero capacity factor. In both cases we assume that the units have no scheduled maintenance requirements and a 1% forced outage rate, corresponding to interconnection characteristics rather than those of individual generating units.
Compared to the Base Case, two 450 MW CC units otherwise needed in 2012 are not added to the resource plan.
The two 500 MW units provide somewhat higher reliability than the two 450 MW CC units deferred. The lower cost of ENS in the interconnection scenario puts the two cases on an equivalent basis.
6.6.8.2 Costs
Table 6-30 presents the cost savings resulting when the costs of this scenario are subtracted from the Base Case values. The 2025 values are extended to 2041 for consistency with an assumed life of 30 years for the new generating plant.
Table 6-30 New 1,000 MW Interconnection - Cost Savings
| All Costs in Millions of 2005 US$ | New Intercon Parameters |
Year | Operating Costs | Cap- ital Costs | Total Direct Costs | ENS Costs | Total Costs | Genera- tion, GWH | Oper Value, $/MWH | Equiv Cap Fac, % |
Fixed & Var O&M | Fuel |
2005 | 0 | 0 | 0 | 0 | 0 | 0 | 0 | 0 | 0% |
2006 | 0 | 0 | 0 | 0 | 0 | 0 | 0 | 0 | 0% |
2007 | 0 | 0 | 0 | 0 | 0 | 0 | 0 | 0 | 0% |
2008 | 0 | 0 | 0 | 0 | 0 | 0 | 0 | 0 | 0% |
2009 | 0 | 0 | 55 | 55 | 0 | 55 | 0 | 0 | 0% |
2010 | 0 | 0 | 338 | 338 | 0 | 338 | 0 | 0 | 0% |
2011 | 0 | 0 | 226 | 226 | 0 | 226 | 0 | 0 | 0% |
2012 | 10 | 65 | 0 | 75 | 2 | 77 | 3,373 | 22 | 39% |
2013 | 10 | 70 | 0 | 81 | 3 | 84 | 3,585 | 23 | 41% |
2014 | 11 | 82 | 0 | 93 | 2 | 95 | 3,820 | 24 | 44% |
2015 | 12 | 100 | 0 | 112 | 4 | 116 | 4,332 | 26 | 49% |
2016 | 12 | 102 | 0 | 114 | 3 | 118 | 4,355 | 26 | 50% |
2017 | 12 | 101 | 0 | 113 | 4 | 117 | 4,361 | 26 | 50% |
2018 | 10 | 73 | 0 | 83 | 3 | 87 | 3,649 | 23 | 42% |
2019 | 12 | 91 | 0 | 103 | 3 | 106 | 4,330 | 24 | 49% |
2020 | 12 | 94 | 0 | 105 | 2 | 107 | 4,339 | 24 | 50% |
2021 | 12 | 93 | 0 | 105 | 3 | 108 | 4,355 | 24 | 50% |
2022 | 12 | 93 | 0 | 105 | 4 | 109 | 4,358 | 24 | 50% |
2023 | 12 | 93 | 0 | 105 | 3 | 108 | 4,354 | 24 | 50% |
2024 | 12 | 93 | 0 | 105 | 4 | 109 | 4,357 | 24 | 50% |
2025 | 12 | 93 | 0 | 104 | 4 | 108 | 4,359 | 24 | 50% |
2026 | 12 | 93 | 0 | 104 | 4 | 108 | 4,359 | 24 | 50% |
2027 | 12 | 93 | 0 | 104 | 4 | 108 | 4,359 | 24 | 50% |
2028 | 12 | 93 | 0 | 104 | 4 | 108 | 4,359 | 24 | 50% |
2029 | 12 | 93 | 0 | 104 | 4 | 108 | 4,359 | 24 | 50% |
2030 | 12 | 93 | 0 | 104 | 4 | 108 | 4,359 | 24 | 50% |
2031 | 12 | 93 | 0 | 104 | 4 | 108 | 4,359 | 24 | 50% |
2032 | 12 | 93 | 0 | 104 | 4 | 108 | 4,359 | 24 | 50% |
2033 | 12 | 93 | 0 | 104 | 4 | 108 | 4,359 | 24 | 50% |
2034 | 12 | 93 | 0 | 104 | 4 | 108 | 4,359 | 24 | 50% |
2035 | 12 | 93 | 0 | 104 | 4 | 108 | 4,359 | 24 | 50% |
2036 | 12 | 93 | 0 | 104 | 4 | 108 | 4,359 | 24 | 50% |
2037 | 12 | 93 | 0 | 104 | 4 | 108 | 4,359 | 24 | 50% |
2038 | 12 | 93 | 0 | 104 | 4 | 108 | 4,359 | 24 | 50% |
2039 | 12 | 93 | 0 | 104 | 4 | 108 | 4,359 | 24 | 50% |
2040 | 12 | 93 | 0 | 104 | 4 | 108 | 4,359 | 24 | 50% |
2041 | 12 | 93 | 0 | 104 | 4 | 108 | 4,359 | 24 | 50% |
Total | 349 | 2,725 | 619 | 3,693 | 100 | 3,793 | 127,671 | | |
Total NPV | 41 | 318 | 305 | 663 | 11 | 675 | 14,869 | | |
Table 6-31 summarizes the results of the value calculations. The new 1,000 interconnection reduces costs equivalent to the unit values shown in Table 6-31 The values shown are nearly identical to corresponding costs from the screening analysis for a 450 MW CC operating at 50% capacity factor. The Capacity Only Value equals the undiscounted capital costs (corresponding the capital costs at operation) divided by 1,000 MW. Relatively small differences arise due to differences in reliability and treatment of IDC.
Table 6-31 New 1,000 MW Interconnection - Value Summary
| Value of New 1,000 MW Interconnection |
| Operating | Total | Capacity Only |
Energy, $/MWH | 24 | 45 | 20 |
Capacity, $/KW | | | 619 |
These results illustrate how much a utility could afford to pay for the combination of installing a new 1,000 MW interconnection and purchasing energy equivalent to a 50% capacity factor. This analysis ignores the potential benefits of profitable sales from Bangladesh to neighboring countries as well as purchases using the new interconnection.
6.6.9 Summary of Conclusions
From the production simulation and system optimization analysis, we can draw several important conclusions:
6.6.9.1 Base Case
- The optimal mix of new resources is fueled by natural gas, with about 20% of new MW coming from SCGT units and 80% from CC units.
- Reliability is low in 2005 – 2007 because there is not enough time to install any units other than the committed units under way.
- Total costs, including the operating costs of the existing system and committed units, and all costs of new units, are about $1.5 billion in 2008 and increase to as much as $3 billion in later years.
- Fuel costs amount to more than 60% of total costs.
- Natural gas requirements grow from 225 BSCF in 2005 to over 700 BCSF in later years.
6.6.9.2 High and Low Demand Scenarios
- The optimal mix of new resources does not change significantly.
- Costs and fuel requirements follow the same trend as demand.
6.6.9.3 Limited Gas
- The optimal mix of new resources includes steam plants fueled by both domestic and imported coal when natural gas is assumed to be unavailable.
- Costs increase significantly when natural gas is not available.
- Natural gas requirements fall, offset by large increases in the use of both domestic and imported coal.
6.6.9.4 High and Low Discount Rates
- High and low discount rates have very little effect on the optimal resource plan, costs, or fuel requirements.
6.6.9.5 High and Low Cost of ENS
- When the LOLP criterion is applied, high and low cost of ENS have very little effect on the optimal resource plan, costs, or fuel requirements.
6.6.9.6 No Application of LOLP Criterion
- When the LOLP criterion is not applied, the optimal resource plan changes.
− Fewer MW of new units are added, and SCGT form a larger percentage of the mix.
− The optimal resource plans all have lower reserve margins and higher LOLP and ENS than the Base Case.
− The lower the unit cost of ENS, the higher the LOLP and amounts of ENS, and the lower the reserve margin.
- The application of the LOLP criterion leads to higher overall system costs, given the values for the other parameters we are using.
- The application of the LOLP criterion has little effect on fuel requirements.
6.6.9.7 Value Scenario – New 500 MW Generating Unit
- The new unit has value close to the costs associated with the unit it replaces, a 450 MW gas fueled CC operating at about 75% capacity factor.
- The new unit’s operating value is $24/MWH. Its total value is $38/MWH assuming operation at about 75% capacity factor.
6.6.9.8 Value Scenario – New 1,000 MW Interconnection
- The new interconnection has value close to the costs associated with the units it replaces, two 450 MW gas fueled CC operating at about 50% capacity factor.
- The new interconnection’s operating value is $24/MWH. Its total value is
$45/MWH assuming operation at about 50% capacity factor.
6.7 RECOMMENDATIONS
We propose the following recommendations based on the results of the screening analysis and the production simulation and system optimization analysis.
Our general recommendation is to follow the Base Case as the framework for planning and future development of the power system. This least-cost plan is based on our current view of the most likely values for the key input parameters.
We recommend below that the PSMP should be updated on a yearly basis. In other words, a new Base Case would replace the one contained in this document. For example, if no new gas reserves were found in a timely manner, a scenario similar to the Limited Gas scenario of this report would probably become the new Base Case.
We offer other recommendations in four general areas.
6.7.1 Eliminate Load Shedding
The most urgent need is to expand the generation and transmission systems to eliminate the routine load shedding that has prevailed for the last ten years and is forecast to continue for the next few years at least. Five recommendations address that critical issue:
- Construct and place in operation the committed generation units as quickly as possible.
- Construct and place in operation the committed transmission projects as quickly as possible.
- Secure financing for committed projects whose financing is not certain.
- Initiate the process leading to implementation of two new 150 MW SCGTs by 2008 and at least one 450 MW CC by 2009 and two such units by 2010.
- Initiate the process leading to implementation the transmission plan for 2010.
6.7.2 Near-Term Strategy
The recommended near-term strategy includes the following elements:
- Use natural gas fueled SCGT for peaking duty and CC for mid-range and base load service as the primary, and possibly exclusive, resource plan additions as long as the resource base indicates sufficient gas to supply them.
- BPDB and PGCB should develop a realistic investment plan concerning planned generation and transmission, in consultation with the GOB and donors.
- Use the Base Case demand forecast for planning purposes until a new demand forecast is developed.
- Use a liquid fuel based natural gas price for purposes of resource plan analysis. This should not be used for pricing gas sales to generators or setting prices to IPPs unless that is decided as a policy of the GOB.
- Undertake a study of the joint least cost development of the natural gas and electric transmission systems.
6.7.3 Assure Natural Gas Availability
The generation expansion plan is based nearly entirely on the premise that natural gas will continue to be available. Assuring that this premise continues to apply is critical.
- Monitor the status of natural gas reserves and deliverability as part of routine electricity planning activities.
- Take the needs of the power sector into account in planning the development of gas fields and transport systems.
6.7.4 Reduce Uncertainty
Many near-term, mostly low-cost study activities will reduce risks and uncertainties, and improve both near- and long-term planning results.
- Update the PSMP on a yearly basis. BPDB and PGCB have the updated planning models and experience to do this with little or no assistance from consultants.
− Some training of BPDB and PGCB in the use of their new models and some of their special applications would be beneficial.
- Monitor the cost and performance worldwide of larger units such as the 700 MW CC in order to gain confidence that they would be suitable for Bangladesh.
- Evaluate the need for application of the LOLP criterion.
- Develop a Bangladesh-specific estimate of the most appropriate value for unit cost of ENS.
- Develop an information base for the cost of domestic and imported coal in Bangladesh.
- Develop a better data base to support dynamic analysis of the transmission system, including data on IPP generators.
- Encourage broader use of power from private generators such as co-generators and self-generators.
− Establish procedures to guarantee ability to safely interconnect and for backup power from the grid.
− Rationalize pricing.
- Conduct more detailed analysis of the value of new resources to support decision- making in specific cases.
Section 7 Transmission Expansion Plan
7.1 APPROACH
7.1.1 Study Objectives
The major objective of the transmission study section of the Master Plan is to review Bangladesh’s 1995 Power System Master Plan and to formulate a transmission plan for the PGCB grid for the period 2005-2025. The secondary objective is to train PGCB engineers in transmission planning with the use of state-of-the-art analytical tools for power system analysis. CYME International T&D software was use to conduct power system analysis associated with the transmission planning process.
7.1.2 Overview of Approach
We applied an approach that was used successfully in similar master planning projects for countries such as Saudi Arabia, Thailand, Malaysia, and the Philippines. This approach comprised the following aspects:
- Delineation of planning criteria
- Development of analytical models for simulation
- Development of horizon year (last year of study period) models and plans
- Staging of plans
- Integration of the master plan
Our first step in the process of conducting the transmission studies for the Master Plan was to establish the planning criteria to be used in the study to represent the desired levels of reliability from the future transmission network. These criteria were documented from discussions with PGCB and Power Cell planning staff and augmented with the study team’s experience.
Once the criteria were established, data bases were developed that define the reference transmission system configuration and a structural model with which the transmission plans can be developed. The data bases comprise:
- Load flow models
- Short circuit model
- Dynamic simulation model
The load flow models, or “cases,” consist of existing, committed and funded transmission circuits, transformers and shunt capacitors, existing and proposed substations with forecasted load and existing and proposed generators dispatched in a manner as to stress the transmission network. For planning purposes, we use a “stressed” dispatch to determine the capability of the planned transmission network to withstand extreme, but probable, operating conditions. The stressed dispatch is distinct from an economic dispatch wherein the system model is for a normal utilization of the network. The initial data base was provided by PGCB in the form of a 2010 load flow case. This initial 2010 model, and the future cases representing study years 2015, 2020, and 2025, were further developed by the study team, incorporating forecasted load and planned generation (from the generation expansion plan).
The generation scenario used for the transmission master plan was the Base Case sufficient gas scenario.
The model for short circuit analysis consists of positive sequence parameters for generators, transmission lines, and transformers that can be used for studying three-phase to ground faults.
The model for dynamic simulation consists of generator parameters, excitation system, voltage regulator and turbine-governor controls dynamics intended to capture automatic system response to disturbances in the transient and steady-state stability time frames.
The data bases were complemented with data for costs for major equipment, including costs for transmission lines, transformers, circuit breakers, and shunt compensation. This data was assembled for cost analysis purposes.
Following definition of the load flow, short circuit, and dynamic data bases the subsequent step involved development of transmission plans for the horizon year. System studies involving load flow, contingency analysis, and economic evaluation were conducted to develop the horizon year plans to the same minimum level of reliability defined by the planning criteria. The resulting horizon year plans were then compared economically and technically to identify the best option for long-term transmission development of the PGCB grid.
Once a picture of the long-term development was established from the horizon year plan, the next step was to “stage” the selected horizon year plan. Staging is the process of identifying the sequence and schedule of each component of the horizon year plan from the initial study year, 2005. Staging was conducted in increments of 5 years; hence for years 2010, 2015, and 2020. In the actual process, staging is performed backward in time from the horizon year; thus, the 2020 requirements are identified first, followed by 2015 and then by 2010.
Finally, the plan as developed in the preceding steps was integrated by testing for sensitivities to various uncertainties, primarily from generation dispatch. Any additional reinforcement or expansion requirements indicated by the sensitivity analysis were then included with the master plan.
7.2 PLANNING CRITERIA
The planning criteria described in this section are applicable to transmission planning evaluations of the bulk power transmission network of national electric power transmission companies like PGCB. These criteria have been developed specifically for the Transmission Master Plan Project in Bangladesh. The criteria developed herein are based on planning criteria used in previous PGCB studies and on discussions with PGCB and Power Cell planning personnel. The facilities covered in this document include AC transmission components rated at 132 KV and above.
This section describes the tests to be performed and defines the standards for acceptable performance. The tests and criteria are divided into steady state and dynamic components.
7.2.1.1 General Guidelines
A basic principle of power system planning is that all equipment should be within normal capacity ratings and normal voltage limits when the system is operating with all scheduled elements in service and is not experiencing faults or other abnormal disturbances.
Furthermore the system should be capable of operating within emergency capacity ratings and emergency voltage limits immediately after a disturbance that results in the loss of a single element (N-1).
Normal capacity ratings and voltage limits represent equipment limits that can be sustained indefinitely without increased risk of equipment failure or loss-of-life. Emergency capacity ratings and voltage limits represent equipment operating conditions that can be tolerated for a relatively short period, recognizing that there may be a small increase in the risk of failure or danger to personnel. PGCB practice nevertheless, is to use normal capacity ratings for both normal operating conditions with all lines in service and for emergency conditions after the loss of a single transmission facility. Through this study then the post-contingency or emergency rating used is the normal rating.
Steady state power flow (load-flow) models are used to investigate system adequacy in terms of satisfying capacity ratings and voltage limits. Dynamic (time-domain) models are used to evaluate system stability under specific fault conditions.
The options selected for system expansion will be based on the reinforcements needed to achieve acceptable conditions as defined by the planning criteria, both for steady state and for the more probable N-1 contingencies.
Normal options for system reinforcement include the addition of new transmission circuits, transformers, and voltage/reactive support devices.
A summary of planning criteria covered under the next sections is given in Table 7-1.
7.2.2 Base Case Steady State Test Criteria
For the base case scenario under peak load conditions an AC power flow solution is calculated. No transmission element shall be loaded above normal ratings and all buses shall be within normal voltage limits, as defined in Table 7-1. Furthermore, all generator units shall be dispatched within normal real and reactive power limits.
7.2.3 Single Contingency Steady State Test Criteria
Following any single contingency event, no circuit element shall be loaded above its emergency rating, though as indicated above, for this study the normal rating rather than the emergency rating will be used for single contingency steady state criteria, all buses shall be within emergency voltage limits, and no loss of load shall occur.
No corrective operator action is allowed when determining whether emergency (for this study normal rather than emergency thermal ratings are used for transmission lines and transformers) limits have been violated. Normal, fast acting automatic control and switching (e.g. reactive support from generators) shall be represented. On-load tap changing shall not be considered a fast acting control option.
Table 7-1 Summary of Transmission Planning Criteria
Acceptable Limits | Allowable Resolution |
Normal Conditions |
Transmission line loading: <100% (new) | Line reinforcements |
Transformer loading: <100% (new) | Transformer additions |
Steady-state voltage range: +/- 5% | Reactive power dispatch or switched shunt additions |
Single Outage Contingencies |
Transmission line loading: <100% | Line reinforcements |
Transformer loading: <100% | Transformers additions |
Steady-state voltage range: +/- 10% | Reactive power dispatch or switched shunt additions |
Transiently stable to 3-phase to ground fault with normal clearing | Faster excitation systems, transmission reinforcements, other countermeasures |
7.2.4 Dynamic Test Criteria
Stability tests shall be performed to ensure that the system shall exhibit transient (first swing) stability from the initial disturbance until a new steady-state condition is attained.
Loads shall be represented with the best available voltage dependent models. For the Master Plan studies, the model shall be: constant current for real power loads and constant impedance for reactive power loads. These models can be applied until better information becomes available on load characteristics.
The stability test shall include test of faults near the largest generator stations and other points in the system that, by engineering judgment, can be determined to constitute a severe testing for system stability. Peak load conditions shall be studied unless engineering judgment indicates other conditions would be more severe. Normal fault clearing time shall be assumed to be 6 cycles for equipment in place as of the time of the Master Plan study and for new equipment rated at or below 400/230 KV.
The system should maintain stability without load shedding, generator dropping, or special remedial actions either automatic or operator-actuated.
7.3 PLANNING METHODOLOGY
This section provides more details on the planning methodology that was first presented in the earlier section on Overview of Approach.
The key to the planning methodology for this master planning study is the development of robust horizon year plans. The horizon year represents the furthest out into the future that we are able to project without losing accuracy due to inherent forecast uncertainty. It also represents a long-term view, essential to a transmission master plan since the components of the plan, such as transmission lines and transformers, have lifetimes of two or three times the horizon year period. Having this time frame for study ensures that the selected components of the plan have long-term application. In contrast to methods that incrementally plan from the initial year at say 5 year intervals, the horizon year method avoids providing for
transmission reinforcements that have a short-term or transitory basis. For example, projects that are needed only for study years 2010 and 2015 but not thereafter fall into this category.
By looking at multiple options to meet the horizon year planning criteria, we are able to define a robust plan that will fit into multiple futures. By analyzing sensitivities such as alternate dispatches, we are able to further enhance the robustness of the plan.
Having established the importance of the horizon year plan, it is equally as important to have an accurate horizon year model. The horizon year model includes the following:
- Horizon year substation peak load forecast
- Horizon year generation plan and peak load dispatch schedule
- A model of existing, committed and funded transmission components such as transmission lines, transformers, substations and switched shunts that comprise the so-called “skeleton” system; i.e., the system that exists today or in the near- term carried forward to the horizon year
Before going into the planning process, we discuss each of these aspects of data in further detail in the following sections.
7.3.1 The Horizon Year Model
7.3.1.1 Horizon Year Substation Load Forecast
Any transmission planning methodology requires a substation load forecast for the planning years (2010, 2015, 2020, and 2025) and corresponding generation scenarios as a starting point. The horizon year approach used for the Master Plan is not an exception.
As indicated above, modeling substation loads in terms of both active power in MW and reactive power in MVAR is of fundamental importance in transmission planning studies. The calculation of substation loads for this study is based on Nexant’s peak regional load forecast developed for the Master Plan time period of 2005-2025, for the five regions in Bangladesh: Dhaka, Southern, Central, Western, and Northern.
An earlier 2010 load forecast by region and by substation developed by PGCB was modified to take into account the Master Plan load forecast for the same year 2010. Based on information provided by PGCB planning staff, substation loads in northern Dhaka and in the irrigation area of the Northern region were adjusted to reflect a likely higher load growth rate. Other substation loads in these two regions, Dhaka and Northern, were then scaled down so that the total demand in these regions equaled the regional demand forecast determined by the Master Plan.
The regional and substation load forecasts obtained in the process described in the previous paragraph were used to calculate the percent of total regional load corresponding to each individual substation in 2010. We assumed that these percents of total regional load distributions would continue until 2025. Using the Master Plan regional load forecast for 2025, we calculated the demand at individual substations by multiplying total regional demand by the percent of total regional load distribution for each substation. The outcome of this process was the substation load forecast for 2025. We obtained substation load forecasts for 2020 and 2015 in the same way as in 2025.
Since we forecast load only as active power in MW, the allocation of reactive power was obtained by assuming a 0.9 power factor for each substation.
Information concerning load power factor obtained for April 17 of fiscal year 2005 indicates that during peak hours a substantial majority of power factors were in the range of 0.85 to
0.95. Based on this data and on discussions with BPDB and PGCB, a 0.9 power factor was used in this study for the load at all substations modeled in the study years of 2010, 2015, 2020, and 2025. This corresponds to an assumption that improvements in distribution system design, equipment, and tariff structure will improve power factors in the distribution systems by 2010 such that a power factor of 0.90 or more can be achieved at each substation.
7.3.1.2 Horizon Year Generation Plan and Peak Load Dispatch Schedule
Generation dispatches were based on power plants determined in the generation planning process of the 2005 Master Plan. In the simulations, the generator schedules were selected such as to stress the transmission system. Operating economics were a secondary consideration in the dispatches.
A base case generation dispatch with as many new generating facilities as possible dispatched to maximum output was developed. To accomplish the schedule of this base case dispatch scenario, existing generating facilities were initially modeled as out of service. Sensitivity analysis was later conducted to test the robustness of the master plan when the existing generation facilities that were off-line in the base case scenario were dispatched to maximum output to test the capability of the resulting transmission plans to deliver total capability of the new and existing generation facilities into the transmission system.
The horizon year dispatch schedule is discussed in further detail in Section 7.4 Basic Generation Dispatch.
7.3.1.3 Horizon Year Skeleton Transmission System Model
For the Master Plan study, the base year is 2010 and the horizon year is 2025. PGCB provided a 2010 base case used for their internal transmission planning studies. This case was modified to include only existing, under construction and funded transmission projects, and proposed new substations intended to relieve nearly overloaded existing substations.
The transmission system defined in this base case, in combination with the horizon year load forecast and generation dispatch comprised the horizon year model.
The base case provides a structural model called the skeleton system with which the transmission plans can be developed in the horizon year. This includes system data for existing system facilities and committed facilities as follows:
- A skeleton transmission system that includes existing, under construction and funded transmission circuits, transformers and power conditioning units (capacitors, reactors, if any) for the base year. Data includes electrical parameters and line and equipment ratings.
- The substation peak load forecast for horizon year.
- Generation dispatches based on generation development for the horizon year.
- Data for fault analysis accompanying each load flow data base.
DESIGN FOR VOLTAGE/REACTIVE CRITERIA | | REACTIVE COMPENS |
| |
- Data for dynamics simulation. At present PGCB does not have a dynamics data base of its power system. A data base for the horizon year was developed for the purpose of the Master Plan. Equipment modeling for CYME dynamic simulation comprising as a minimum generator parameters, excitation system and voltage regulator controls, and turbine-governor controls was developed using typical data.
- Costs for major equipment, including costs for transmission lines, transformers, and breakers as provided by PGCB, and typical cost for shunt compensation.
7.3.2 Horizon Year Planning Method
Horizon year plans are developed on the basis of load flow (steady-state) and dynamic simulation studies. The initial procedure assumes a decoupling of the requirements for thermal capacity, voltage compensation, and stability. However, it is recognized that these studies are not independent and it is necessary to structure the studies so that the interdependence of solutions to steady-state and dynamic problems is recognized.
For the Master Plan study, the procedure followed to develop and verify transmission plans for the horizon year is as shown in Figure 7-1.
HORIZON YEAR BASE CASE DESIGN FOR THERMAL CAPACITY TRANSMISSION ADDITIONS ATION, CONTROLS TEST WITH STEADY-STATE CRITERIA DESIGN FOR STABILITY |
TEST WITH DYNAMIC CRITERIA | |
|
| STABILITY MEASURES |
| | |
TRANSMISSION ALTERNATIVE
Figure 7-1 Horizon Year Development Process
7.3.2.1 Planning for Thermal Capacity
In preliminary design for thermal capacity of the transmission system in the horizon year, the interactive process incorporates planning judgment in the selection of transmission additions like new transmission lines and transformers needed to comply with the planning criteria that no transmission element shall be loaded above normal ratings as defined in Table 7-1.
7.3.2.2 Determining Preliminary Reactive Compensation
Once the planning process for thermal capacity indicates compliance with planning criteria, load flow studies initially identify equipment requirements, like shunt capacitors, for voltage control to comply with the planning criteria that all buses shall be within normal voltage limits, as defined in Table 7-1.
7.3.2.3 Contingency Analysis, Testing Performance for N-1 Conditions
The transmission alternatives are also designed to perform within criteria on loss of any single element. Contingency analysis considers the loss of any single line or transformer (N- 1 criteria) and their potential impact on transmission loading. Contingencies that result in criteria violations are identified. Additional transmission facilities like new transmission lines, transformers, and shunt capacitors needed to comply with the N-1 planning criteria are also specified. The planning criteria for this study specify that the system equipment shall be within normal ratings (as indicated by PGCB) following any N-1 contingency.
7.3.2.4 Stability Tests
The transmission plan is tested in accordance with stability criteria. This task is confined to testing transient as opposed to dynamic stability. The reason for this constraint is that dynamic conditions do not govern the requirements for transmission and major equipment.
Single line-to-ground faults were specified in the dynamic criteria, but three-phase faults were applied when testing for dynamic response of the plans. Although three-phase faults are relatively rare events they have been applied in PGCB planning to represent the effect of extreme weather conditions and to provide some margins to compensate for uncertainties and unknowns (load characteristics, machine parameters, etc.)
7.3.2.5 Short Circuit Analysis
Short circuit studies are performed on the selected transmission plan for the horizon year. Three-phase to ground faults are applied to all buses 132 KV and above. Though three- phase faults are less likely to occur than single line to ground faults, they are in general more severe and thus produce more conservative results.
7.3.3 Economic Evaluation of Transmission Plans
In addition to considering the technical characteristics of a transmission plans, the transmission planner needs to take into account the economic characteristics associated with them. The transmission plans developed for Bangladesh’s bulk transmission system for the horizon year of 2025 are evaluated from the economic point of view. The economic evaluation considers capital costs of transmission lines, transformers, and capacitors as well as the cost of transmission losses and operation and maintenance.
The cost of losses is estimated from the peak load MW loss using an empirical formula based on system load factor. First the average hourly loss is calculated using:
Apl = Plpeak x (0.3 x LF + 0.7 LF2) Where:
Apl = average hourly loss in MW Plpeak is the peak load loss in MW LF is the system load factor
The average hourly loss is converted into energy by multiplying by the duration, say one year. This is subsequently converted into cost by multiplying by the cost of losses per MWH.
The economic comparison is based on a net present value calculation with specified discount rate. 2005 prices (discussed in Section 5) are used with no escalation.
7.3.4 Back Staging of Transmission Plan
Back staging, to determine the time during the planning period when the components of the transmission plan developed in the horizon year will be needed, is conducted on five year intervals from the horizon year 2025 down to the initial study year. The objective is to identify when each of the components of the horizon year is needed to meet planning criteria.
The analytical process is very similar to that used for the horizon year planning process, however, the focus is on identifying which planned projects are not needed to meet criteria for each staging year. As much as possible, the process avoids having to identify new transmission projects that are needed only in staging years. Such projects represent transitory requirements that are best addressed by operating measures such as re-dispatch or special protective schemes. Otherwise, they would represent an investment that would become unutilized by the horizon year.
7.4 BASIC GENERATION DISPATCH
Section 2 describes the existing and committed generation and transmission systems. Section
A.4 in Appendix A provides the substation load forecast through 2025. Section 6 provides the generation expansion plan through 2025. In this section we present the dispatch of the generation existing in each of the study years used in the transmission system analysis.
The schedule did not provide time for analysis of many different scenarios for generation dispatch. Thus we needed to determine a dispatch that would stress the system in a manner likely to address the most important issues.
The existing system can accommodate the maximum output of existing units. Therefore, our first objective was to provide the ability of the system to handle maximum dispatch of new (post-2005) generation. A secondary objective was to maximize Dhaka generation, which would provide for maximum transfers out of the region with the largest excess of generation over regional load.
Consistent with these objectives, we established the following dispatch principles:
1) Provide for 5% spinning reserve.
2) First dispatch all new units at 100%.
3) If 2) provides more generation than required by the level of demand, reduce generation and carry spinning reserve outside Dhaka. Dispatch to maximize transfers into the most deficient region, which is usually the Southern region.
4) If 2) provides less generation than required by the level of demand, dispatch existing units in Dhaka first, then outside Dhaka to maximize transfers into the most deficient region. Carry spinning reserve outside Dhaka to maximize transfers.
Table 7-2 summarizes the resulting generation dispatch for each study year. The table entries are net MW into the grid at the high side of the power plant main transformers, before transmission losses to the 132 KV substations where power is delivered to the distribution entities. Section B.1 in Appendix B provides detail on the dispatch of each unit at each power plant for all cases.
Table 7-2 Summary Generation Dispatch for All Cases
| MW DISPATCHED AND MW DEMAND FOR CASES |
REGIONS | 2025 Base | 2020 Base | 2015 Base | 2010 Base |
Dhaka Region Total Dispatch | 9,909 | 6,677 | 4,841 | 4,077 |
Regional Demand | 9,071 | 6,573 | 4,597 | 3,104 |
Central Region Total Dispatch | 1,238 | 1,088 | 488 | 283 |
Regional Demand | 1,656 | 1,200 | 839 | 567 |
Southern Region Total Dispatch | 2,738 | 2,850 | 1,700 | 800 |
Regional Demand | 3,944 | 2,857 | 1,998 | 1,349 |
Northern Region Total Dispatch | 2,330 | 1,880 | 1,410 | 1,001 |
Regional Demand | 2,329 | 1,687 | 1,180 | 797 |
Western Region Total Dispatch | 3,097 | 1,497 | 1,347 | 447 |
Regional Demand | 2,312 | 1,675 | 1,172 | 791 |
| | | | |
National Total Dispatch | 19,312 | 13,992 | 9,786 | 6,608 |
National Demand | 19,312 | 13,992 | 9,786 | 6,608 |
Sensitivity analysis was conducted for years 2025 and 2020 by modifying the dispatch shown in Table 7-2. The sensitivity analysis was conducted to test the capability of the transmission plan to evacuate the system generation without violation of criteria. A more detailed explanation of the process followed to conduct the sensitivity analysis is available in Sections
7.5 and 7.8.
7.5 HORIZON YEAR 2025 PLANS
Two main options for expansion have being considered in this study: Plan A, which includes a committed 400 kV transmission facility in the Dhaka area and additional 230 kV and 132 kV facilities; and Plan B, which in addition to the committed 400 kV facility in the Dhaka
area considers additional transmission facilities ranging from 132 kV to 400 kV voltage levels. Other options, such as Extra-High Voltage transmission and direct-current transmission, did not offer technical or economic features that would compete with the main options.
Using load flow and contingency analysis techniques, the two transmission plans mentioned above were developed. Both plans were developed to meet planning criteria in the horizon year 2025. The process by which this analysis is conducted is described in detail in Section 7.3.2, Horizon Year Planning Method.
Figure 7-2 shows the 230 KV and 400 KV components of Plan A for the horizon year transmission system. The load flow data base used for load flow and contingency analysis in the horizon year is included in Appendix B.
Figure 7-2 One-Line Diagram of Plan A for Year 2025 (On following page)
2042 BRPUK23
1.031
-211.01 2052 SRIPR23
-4.4
-64.07
4.59
-64.07
4.59
230.00
71.79
89.54
9.59
100.00 %
89.54
9.59
100.00 %
89.54
9.59
100.00 %
89.54
9.59
100.00 %
1442 BARPUKR
1.025
-9.1
2039 KASIM23
1.012
-6.5
2011 TONG23
0.997
-5.0
-92.76
5.70
-92.76
5.70
642.73
413.77
2010 GRSL23
0.998
-4.0
-82.38
82.98
-8.29
-211.01
-8.29
1.022
-2.6
140.11
4.85
100.00 %
140.11
4.85
100.00 %
140.11
4.85
100.00 %
1146 SRIPR
1.022
-6.0
145.19
8.61
97.50 %
145.19
8.61
97.50 %
2040 BOG23
1.027
-2.3
64.42
-25.68
1440 BOGNW
1.008
117.88 -5.2 46.39
100.00 %
117.88
46.39
127.25
-190.88 -87.28
191.40
-153.16
191.40
-153.16
-290.37
230.51
-290.37
230.51
98.97
-77.35
291.99
-222.57
291.99
-222.57
102.42
77.79
-51.10
-82.38
-51.10
87.84
40.47
95.83 %
1125 TONGI 87.84
47.73
82.98
47.73
145.19
8.61
97.50 %
145.19
8.61
97.50 %
64.42
-25.68
100.00 %
154.29
-190.88
105.00 %
105.00 %
98.97
95.00 %
102.42
1.017 40.47
95.83 %
-7.5
-0.84
0.84
1030 COMI-N
145.19
8.61
97.50 %
-241.23
3.58
117.88
46.39
154.29
127.25
-87.28
-77.35
105.00 %
77.79
95.00 %
87.84
40.47
-14.19
1.02
1.021
-11.5
-241.23
100.00 %
105.00 %
95.83 %
-0.84
0.84
3.58
2050 KABIR23
1.019
-7.3
127.25
-87.28
1139 KASIM
1.000
102.42
77.79
95.00 %
87.84
40.47
700.00
220.95
-14.19
160.83
1.02
2036 SRJGNJ
105.00 % 1126 KABPR
1.013
-9.2
-342.98
95.83 %
16.56 -158.63
-13.62
111.98
-20.51
1.040
244.24 2.5
-10.6
-24.07
-342.98
102.42
77.79
87.84
40.47
1130 GHRASL
160.83
16.56
-158.63
-13.62
111.98
-20.51
1.76 213.81
-24.07 95.00 %
95.83 %
1.018
71.36
244.24 9.11
-7.0
11.74
1201 ASHUGJ
1.76
-8.05
59.38
-8.05
59.38
8.17
213.81
9.11
30.49
450.00
111.30
450.00
111.00
1120 MIRPUR
1.017
-6.4
119.04
27.14
97.50 %
119.04
27.14
97.50 %
119.04
102.42
77.79
95.00 %
102.42
77.79
95.00 %
85.19
87.84
40.47
95.83 %
87.84
40.47
95.83 %
103.86
2008 ASHU23
1.020
-2.6
100.00 %
71.36
11.74
100.00 %
71.36
11.74
100.00 %
1.012
-6.1
-316.33
12.61
2001 RAJN23
0.998
-15.4
-67.55
8.17
-67.55
-38.66
-3.56
100.00 %
30.49
-3.56
27.14
97.50 %
119.04
27.14
-84.89
10.27
-84.89
10.27
-12.73
85.19
-12.73
93.12
103.86
93.12
1104 MANKNG
133.91
63.63
-316.33
12.61
2005 COMI23
0.997
-0.00
-0.00
-0.00
-0.00
71.11 100.00 %
97.50 %
343.65 99.21
1123 UTTAR132
1.010
81.63
10.29
-7.9
-38.66
71.11
1412 BAGBRI
2034 AMIN230
1.008
-2.02
20.93
97.50 %
1.016
-6.5
141.67
24.38
-7.4
92.50 %
1003 HATHAZ
1.015
97.50 %
-109.88
2030 BAGHA230
1.026
2.8
1.028
2.0
-2.4
345.35
373.92
63.43
-372.79
-57.76
321.10
32.37
321.10
-320.64
-30.39
-320.64
99.21
97.50 %
141.67
133.91
81.63
92.50 %
-18.5
63.63
10.29
12.14
-109.88
1015 BARAU132
38.97
-80.95
38.97
-80.95
2020 ISHRDI
95.16
-19.94
95.16
-19.94
96.55
450.00
217.06
35.12
345.35
35.12
373.92
63.43
373.92
63.43
180.92
-372.79 32.37
-57.76
-372.79
-57.76 2023 MIRPU23
1.001
-3.5
-180.59
-30.39
20.93
97.50 %
99.21
20.93
97.50 %
-212.51
-36.56
-212.51
-36.56
24.38
97.50 %
141.67
24.38
97.50 %
133.91
81.63
92.50 %
133.91
81.63
-103.11
-97.33
-103.11
-97.33
97.50 %
63.63
10.29
97.50 %
12.14
2002 HATZ23
0.997
-15.4
0.00
-4.58
0.00
1.012
-18.4
101.98
52.04
95.83 %
1.005 -1.54
3.6 100.00 %
1401 ISHRDI
1.007
450.00
96.82
180.92
-96.66
-180.59
90.29
48.33
2003 MANKNG23 92.50 %
63.63
-4.58
142.02
-141.75 101.98
-278.95
1.3
217.06
96.82
-96.66
96.25 %
0.993
-298.62
150.00 10.29
89.86
-90.48
52.04
103.20 96.55
4034 AMIN400 191.81
180.92
-180.59
90.29
2022 UTTAR23
0.999
213.11
40.37
-3.9
133.91
81.63
-147.56
44.52
97.50 %
142.02
-141.75
95.83 %
-278.95
103.20
2044 BMARA23
-1.54
100.00 %
0.943
0.5
159.83
90.00 %
96.82
103.84
87.90
-96.66
1134 AMINBAZ
48.33
96.25 %
-4.0
213.11
40.37
394.00
92.50 %
133.91
-298.62
-147.56
366.00
134.79
89.86
-106.26
20.80
-90.48
-11.22
12.42
-11.22
101.98
52.04
95.83 %
1.002
4.5
279.65
-100.50
96.55
-1.54
100.00 %
191.81
159.83
90.00 %
95.00 % 1.025
-4.8
103.84
87.90
90.29
48.33
96.25 %
240.08
450.00
81.63
92.50 %
450.00
139.71
-106.26
20.80
-106.26
20.80
12.42
279.65
-100.50
-383.61
191.81
159.83
95.00 %
103.84
90.29
48.33
175.09
208.88 -208.81
1107 RAMPRA
1.015
-8.5
2016 RAMP23
0.969
-5.3
366.00
134.79
106.35
2025 BARAU23
0.990
-15.9
450.00 2032 KHUL23 1.035
1332 KHULNW
-319.64 90.00 %
87.90
95.00 %
96.25 %
2004 SIDGJ230
1.000
123.15 -122.98
208.88
301.07
78.00
-22.10
106.35
123.91
65.84
30.13
150.00
10.05
15.1
132.33
23.65
100.00 %
1.027
12.0
-383.61
-319.64
191.81
159.83
90.00 %
103.84
87.90
95.00 %
90.29
48.33
2045 OLD230 96.25 %
-2.9
123.15
151.66
-208.81 161.43
-122.98 301.07
161.43
44.52
450.00
139.71
-22.10
106.35
-22.10
11.22
-15.07
11.22
-15.07
95.83 %
1310 BMARA
110.09
78.55
-109.29
1.001
1101 HARIPR
81.19
-0.00
200.38
123.91
1.017 95.00 %
1360.00
40.60
103.84
-2.9
90.29
1.018
95.00 %
-0.22
75.64
264.62
-262.90
65.84
1.4
110.09
78.55
95.00 %
321.98
450.00
132.33
23.65
100.00 %
-109.29
40.60
87.90
95.00 %
-329.55
-40.84
48.33
96.25 %
333.01
1145 OLDAIR
1.020
-5.0
-6.6
151.66
81.19
95.00 %
-0.00
-0.22
2015 HAR360
200.38 54.38
75.64
200.38 264.62
75.64 54.38
-48.94
-262.90
-48.94
197.56
84.31
197.56
-197.09
-83.69
-197.09
95.83 %
123.91
65.84
-89.75
116.78
55.91
700.00
0.998
200.38 264.62
-262.90
84.31
-83.69
95.83 %
26.80
-89.75
26.80
132.33
23.65
100.00 %
-329.55
-40.84
-329.55
333.01
55.91
333.01
226.41
700.00
151.66
81.19
95.00 %
-311.54
-161.19
-3.0
312.68
166.57
75.64
54.38
264.62
54.38
-48.94
-262.90
-48.94
84.36
23.37
97.50 %
2024 KULSH23
2049 JHNDHA23
197.00
0.98
-40.84
109.53
55.91
226.41
-311.54
-161.19
312.68
166.57
2026 MD-SKL23
84.36
0.989
-15.8
1013 KULSH132
1.005
0.994
6.8
132.33
JHNDHA132 3.65
-43.63
109.53
700.00
226.41
2029 SHMPR23
151.66
81.19
-311.54
-161.19
312.68
166.57
1.012
-12.7
23.37
97.50 %
-18.9
99.56
32.15
1.004
3.8
100.00 %
141.64
-43.63
700.00
122.89
1.008
-2.0
95.00 %
-311.54
312.68
-199.65
-74.95
84.36
97.50 %
99.56
132.33
23.65
95.53
95.00 %
141.64
-356.99
-88.12
-356.99
360.19
103.59
360.19
226.41
-19.37
103.33 %
151.66
81.19
95.00 %
-161.19
2012 HARI23
166.57
321.18
7.97
321.18
-199.65
-74.95
23.37
97.50 % 1005 MADNHT
1.011
32.15
97.50 %
100.00 %
95.53
95.00 %
-88.12
103.59 2006 MAWA23
1.029
122.89
-19.37
151.66
0.998
-3.0
7.97
-199.65
-74.95
84.36
23.37
-17.6
132.33 141.64
-356.99
-88.12
360.19
103.59
1.6
103.33 %
81.19
95.00 %
-199.65
-74.95
97.50 %
99.56
32.15
23.65
100.00 %
95.53
95.00 %
-356.99
-88.12
360.19
103.59
122.89
-19.37
156.08
60.59
96.25 %
2007 MADNHT23
97.50 %
99.56
32.15
132.33
23.65
100.00 %
141.64
95.53
95.00 %
-356.99
-88.12
360.19
103.59
103.33 %
1129 SHAMPR
-28.69
-37.31
151.66
81.19
95.00 %
28.71
34.05
450.00
217.07
156.08
60.59
96.25 %
0.997
-15.1
90.30
-38.77
90.30
-38.77
97.50 %
295.89
49.30
295.89
49.30
244.45
19.47
244.45
19.47
1113 HASNBD
1.019
-4.9
141.64
95.53
95.00 %
287.31
110.79
287.31
110.79
141.52
-85.68
141.52
-85.68
2019 SITLA230
0.986
-5.3
1114 SITLA132
108.34 0.997
-20.37 -5.4
-141.30
85.03
-141.30
85.03
-28.69
-37.31
-28.69
-37.31
1112 MADAN132
4014 MEGH400
0.956
215.85
88.82
92.50 %
28.71
34.05
28.71
34.05
450.00
260.07
2014 MEGH23
1.012
156.08
60.59
96.25 %
156.08
60.59
96.25 %
70.58
1323 BAGHBRI 2.66
-241.52
0.995
100.83 %
2018 MADAN23 118.60
1.008
1.3
215.85
-1.9
156.08
1320 BARISL
1.031
1.017
8.7
100.00 %
-13.75
2013 HASN23
1.005
-2.6
108.34
-20.37
0.991
-2.8
95.00 %
-5.7
700.00
88.82
92.50 %
60.59
96.25 %
6.3 89.95 2053 BARIS23
70.58
-241.52
-1.6
100.83 %
118.60
338.07
215.85
450.00
10.42 1.008
2.66
100.00 %
-13.75
84.81
95.00 %
700.00
88.82
156.08
60.59
216.75
97.50 % 8.4
-286.41
178.07
-177.90
118.60
338.07
92.50 %
1006 SIKLBHA
96.25 %
2009 SIKBHA23
89.95
10.42
97.50 %
89.95
-134.93
70.58
2.66
100.00 %
135.65 2051 BAGHER23
-107.10
-286.41
-107.10
127.48
178.07
127.48
-127.21
-177.90
-127.21
84.81
95.00 %
450.00
217.07
385.37
159.79
215.85
88.82
92.50 %
215.85
88.82
1.012
-18.4
156.08
60.59
96.25 %
156.08
1.000
-13.8
10.42
97.50 %
-15.63
-134.93
-15.63
9.76
135.65
9.76
1.017
10.5
385.37
159.79
92.50 %
60.59
96.25 %
7.5.1 Load Flow and Contingency Analysis Results
Since the initial load flow data base utilized in the horizon year for load flow and contingency analysis includes only the existing 2005 transmission system facilities and those committed projects provided by PGCB, many thermal overloads and voltage violations were initially detected during these analyses.
New transmission facilities needed to transfer power from generation sites identified by the generation planning process were identified in the horizon year. These facilities include:
- Transmission lines from the Mawa generation site located southwest of Dhaka to the Hasnabad and Aminbazar substations located in the western side of Dhaka city.
- Transmission lines from the Mandahat/New Sikalbaha generation site to Mandahat and Sikalbaha substations located in the Chittagong area in the Southern region of Bangladesh.
A substantial number of overloads and voltage violations, throughout the transmission grid, resulted during the load flow and contingency analysis. These led to new transmission lines that would best relieve the violations.
- In the Dhaka area severe thermal overloads, and their resolving transmission additions, were identified in:
− Tongi-Uttara-Mirpur-Aminbazar 132 KV path and the Hasnabad-Sitalakhya- Mandahat 132 KV path. These paths can be identified in Figure 2-1 (Section 2). Upgrade of existing 132 KV substations at Uttara, Mirpur, Madanganj and Sitalakhya will be required to accommodate the proposed 230 KV facilities.
− Several 132 KV facilities in the northwest portion of the city. To relieve these overloads two 230 KV circuits are proposed, one from Tongi to Kasimpur and a second one from Kasimpur to Kabirpur.
− The existing 230 KV circuits from Meghnaghat to Haripur. Two new parallel 230 KV circuits are proposed between Meghnaghat and Haripur.
- In the Southern region severe thermal overloads, and the corresponding transmission additions, were identified in:
− The 132 KV ring comprised of Hathazari-Baraulia-Kulshi-Mandahat- Hathazari. The ring is proposed to be upgraded from 132 KV to 230 KV. Upgrade of existing 132 KV substations at Baraulia, Kulshi and Mandahat will be required to house the proposed 230 KV facilities. Hathazari 230 KV substation is already in operation.
− The existing 132 KV circuit from Comilla North to Comilla South. Three new parallel 132 KV circuits are proposed between Comilla North and Comilla South.
- In the Western region severe thermal overloads and corresponding additions were identified in:
− Several 132 kV facilities around Khulna. To relieve these overloads several 132 kV circuits are proposed from Khulna Central 132 kV to Khulna New 132
kV substations and two new 230 kV circuits from Khulna new 230 kV to a new Bhandaria 230 kV substation and from the new Bhandaria 230 kV substation to a new Barisal 230 kV substation. Additionally, a tap on the new 230 KV line from Khulna to Ishurdi is proposed to link this line to the Jhenida 132 kV. Upgrades to the Jhenida 132 kV substations are needed to link it to the Khulna to Ishurdi 230 kV line. This tap will assists in relieving overloads under post-contingency operating conditions.
- In the Central region severe thermal overloads and corresponding additions were identified:
− Several 132 kV facilities around Ashuganj. To relieve these overloads one 132 kV circuit is proposed from Joydevpur 132 kV to Mymensingh 132 kV substations.
- In the Northern region several thermal overloads and corresponding additions were identified:
− Two new 132 kV circuits between Bogra 132 kV and Noagaon 132 kV substations are proposed to relieve an overload on the two 132 kV circuits between these substations.
− Three new 132 kV circuit between Shahzadpur 132 kV and Baghabari 132 kV substations is proposed to relieve overload on the existing 132 kV circuit between these substations.
Voltage criteria violations were likewise detected in the analysis of the horizon year. Voltage criteria specify that steady-state voltages, with all equipment in service, should be within a range of +/- 5% of nominal voltage and within a range of +/- 10% of nominal voltage after the loss of a single transmission facility. The load flow and contingency analyses, in the horizon year 2025, uncovered severe voltage violations which were solved by placing shunt capacitors at substations in the 132 kV and 230 kV systems.
The objective of identifying the location and size of shunt capacitors is to determine the level of reactive compensation needed to keep voltages within criteria and to prevent voltage collapse. The final location and size of the capacitors identified by this study could change during implementation, however, the total amount of MVARs included in the Master Plan is indicative of the overall system requirements to control voltage.
More detailed analysis during the implementation stage is fundamental in determining the final location and size of capacitors. Nevertheless, the results of this study as stated above provide the level of reactive compensation needed to keep voltages within criteria and to prevent voltage collapse. Table 7-3 shows the level of reactive compensation by region for the horizon year. This is the same for Plan A and Plan B. The table shows that the level of reactive compensation in general tends to be higher in the major load centers in the country like Dhaka and the Chittagong area in the Southern region.
Table 7-3 Reactive Compensation by Region for 2025
Region | Reactive Compensation (MVAR) |
Southern | 1,285 |
Dhaka | 1,665 |
Central | 360 |
Western | 780 |
Northern | 625 |
Total | 4,715 |
7.5.2 System Summary – Generation Load Losses
Transfer of power from generation to load centers is a key function of the transmission system. As the transfer of power takes place there are associated transmission losses. Transmission losses are of two types: active losses in MW that occur in the resistive elements of transmission lines and transformers in the form of heat dissipation, and reactive losses in MVAR which is power supplied to the electromagnetic fields of transmission lines and transformers for their normal operation. For Plan A, Table 7-4 shows active and reactive generation in MW and MVAR, respectively, active and reactive loads in MW and MVAR, respectively, reactive power from capacitors in MVAR, reactive power due to transmission lines’ capacitive effect in MVAR, and active and reactive losses by region for the horizon year. The total active losses are about 1.7% of total net load while reactive power losses are substantially higher.
Table 7-4 Generation Load and Losses by Region – Plan A
| GENERATION | LOAD | CAPAC- ITORS | LINE CHARGING | LOSSES |
REGION | MW | MVAR | MW | MVAR | (MVAR) | (MVAR) | MW | MVAR |
SOUTHERN | 2738 | 948.5 | 3825 | 1852.1 | 1310.4 | 213.6 | 52.8 | 658.6 |
DHAKA | 9849.4 | 4332.5 | 8799 | 4261.4 | 1513.6 | 745.0 | 127.8 | 2198.4 |
CENTRAL | 1238 | 466.0 | 1606.3 | 778 | 372.5 | 75.6 | 27.9 | 216.8 |
WESTERN | 2907 | 547.9 | 2242.6 | 1086.2 | 816.4 | 234.6 | 69.5 | 612.2 |
NORTHERN | 2330 | 748.2 | 2259.3 | 1093.8 | 649 | 274.5 | 52.1 | 490.7 |
TOTALS | 19062.4 | 7043.1 | 18732.2 | 9071.5 | 4661.9 | 1543.3 | 330.1 | 4176.7 |
7.5.3 Short Circuit Results
In conducting long range planning studies, it is useful to determine short circuit currents to develop an understanding of the level of these currents in the horizon year. Short circuit studies following IEC standards used by PGCB were performed for transmission Plan A. The test was conducted with all generators in service to obtain maximum levels of stress. Table 7- 5 shows current levels for three-phase faults at 230 KV and 400 KV busses. A table including 132 KV, 230 KV and 400 KV buses is included in Appendix B.
Table 7-5 Short Circuit Currents for Plan A at 2025
Bus # | Bus Name | KV Level | 3 Phase(A) | 3 Phase (KA) | 3 Phase (MVA) |
2001 | RAJN23 | 230 | 28893 | 28.893 | 11509 |
2002 | HATZ23 | 230 | 33184 | 33.184 | 13219 |
2003 | MANIK23 | 230 | 36975 | 36.975 | 14729 |
2004 | SIDGJ23 | 230 | 69306 | 69.306 | 27609 |
2005 | COMI23 | 230 | 29880 | 29.88 | 11902 |
2006 | MAWA23 | 230 | 68662 | 68.662 | 27352 |
2007 | MANDAHT | 230 | 35472 | 35.472 | 14130 |
2008 | ASHU23 | 230 | 34595 | 34.595 | 13781 |
2009 | SIKAL23 | 230 | 35466 | 35.466 | 14128 |
2010 | GRSL23 | 230 | 52060 | 52.06 | 20738 |
2011 | TONG23 | 230 | 55150 | 55.15 | 21969 |
2012 | HARI23 | 230 | 74606 | 74.606 | 29720 |
2013 | HASN23 | 230 | 71340 | 71.34 | 28419 |
2014 | MEGH23 | 230 | 83268 | 83.268 | 33170 |
2015 | HAR360 | 230 | 70044 | 70.044 | 27902 |
2016 | RAMP23 | 230 | 45251 | 45.251 | 18026 |
2018 | MADAN23 | 230 | 40003 | 40.003 | 15935 |
2019 | SITLA230 | 230 | 45674 | 45.674 | 18194 |
2020 | ISHR23 | 230 | 27326 | 27.326 | 10885 |
2022 | UTTAR23 | 230 | 52127 | 52.127 | 20764 |
2023 | MIRPU23 | 230 | 58662 | 58.662 | 23369 |
2024 | KULSH23 | 230 | 29882 | 29.882 | 11903 |
2025 | BARAU23 | 230 | 28776 | 28.776 | 11462 |
2026 | MD-SKL23 | 230 | 43096 | 43.096 | 17167 |
2029 | SHAMPUR | 230 | 67235 | 67.235 | 26784 |
2030 | BAGHA23 | 230 | 24420 | 24.42 | 9727 |
2032 | KHUL23 | 230 | 29719 | 29.719 | 11838 |
2034 | AMIN23 | 230 | 74342 | 74.342 | 29615 |
2036 | SJGNJ23 | 230 | 28839 | 28.839 | 11488 |
2039 | KASIM23 | 230 | 35577 | 35.577 | 14172 |
2040 | BOG23 | 230 | 16608 | 16.608 | 6615 |
2042 | BRPUK23 | 230 | 10130 | 10.13 | 4034 |
2044 | BMARA23 | 230 | 27042 | 27.042 | 10772 |
2045 | OLDRPT23 | 230 | 50697 | 50.697 | 20195 |
2049 | JHNDHA23 | 230 | 18432 | 18.432 | 7342 |
2050 | KABIR23 | 230 | 28526 | 28.526 | 11363 |
2051 | BNDRIA23 | 230 | 14116 | 14.116 | 5622 |
2052 | SRIPUR23 | 230 | 22962 | 22.962 | 9146 |
2053 | BARIS23 | 230 | 10637 | 10.637 | 4236 |
4014 | MEGH400 | 400 | 36455 | 36.455 | 25256 |
4034 | AMIN400 | 400 | 31077 | 31.077 | 21530 |
These short circuit levels are useful in specifying future substation equipment, particularly circuit breakers, and substation configurations.
7.5.4 Stability Results
Planning criteria further specify that transmission design meet a minimum capability for responding to large disturbances; i.e., that the design be transiently stable. In this stage of the analysis, the tests for stability were limited to three-phase faults with normal clearing and loss of a circuit during peak load for transmission Plan A. This is sufficient to allow an evaluation of the ability of the transmission plan to maintain stability.
PGCB does not currently have available a dynamic data base for studies which require dynamic simulations. To obtain parameters for generators, excitation systems, and governors used in dynamic simulations it is necessary to review maintenance manuals for individual generating units, but these manuals are usually available only at the generating facilities.
Given the amount of time available to complete the transmission studies it was not possible to visit each generation facility throughout the country to obtain the aforementioned data.
Therefore typical data for the type of equipment mentioned above was used to develop a dynamic data base to conduct the transient stability study. Generator parameters were available for some existing units and these were used in the process of building the dynamic data base.
Active and reactive loads were modeled as a function of voltage. Active loads were modeled as constant current loads, where power is proportional to the voltage at the terminal of the load. Reactive loads were model as constant impedance loads, where power is a function of the square of the voltage at the terminal of the load.
Three-phase faults were applied at several 230 KV buses located near generating stations. Faults were cleared six cycles after application of the faults.
Since stability tests were performed to ensure that the system exhibits transient (first swing) stability from the initial disturbance until a new steady-state condition is attained, the running time used was 2 seconds.
The typical time sequence assumed for contingencies is shown in Table 7-6. All times are given in cycles from the point of inception of the fault.
Table 7-6 Typical Time Sequence for Dynamic Simulations
Typical Time Sequence for 230 and 400 KV Breakers |
Time (cycles) | Switching Sequence |
0 | Fault on bus |
6 | Local non-failed breaker and remote breaker(s) open |
Table 7-7 shows a list of the contingencies considered in the dynamic simulation, their description, and information concerning the results of the simulation, i.e., either S for stable cases and U for unstable cases. The system is stable for all faults considered.
Table 7-7 Three-Phase Faults
ID | Fault Simulation Description | Simulation Results |
NCF-01 | 3-Phase Fault at Ghorasal 230 KV, Open line Ghorasal to Tongi 230 KV | S |
NCF-02 | 3-Phase Fault, at Meghnaghat 230 KV, Open line Meghnaghat to Shampur Tap 230 KV | S |
NCF-03 | 3-Phase Fault at Hasnabad 230 KV, Open line Hasnabad to Aminbazar 230 KV | S |
NCF-04 | 3-Phase Fault, at Comilla N 230 KV, Open line Comilla N to Meghnaghat 230 KV | S |
NCF-05 | 3-Phase Fault, at Hathazari 230 KV, Open line Hathazari to Comilla N 230 KV | S |
NCF-06 | 3-Phase Fault, at Khulna 230 KV, Open line Khulna to Jhenida 230 KV | S |
NCF-07 | 3-Phase Fault, at Bheramara 230 KV, Open line Bheramara to Ishurdi 230 KV | S |
NCF-08 | 3-Phase Fault at Ghorasal 230 KV, Open line Ghorasal to Ishurdi 230 KV | S |
NCF-09 | 3-Phase Fault at Barapukuria 230 KV, Open line Barapukuria to Bogra New 230 KV | S |
NCF-10 | 3-Phase Fault at Comilla N 230 KV, Open line Comilla N to Ashuganj 230 KV | S |
Figures 7-3 and 7-4 show rotor angle plots for contingencies NCF-01 and NCF-04. The rotor angle plots show the rotor angle swings in electrical degrees over the transient period (the figures plot out to 2.5 seconds) for selected generators in various locations of the grid. First swing stability is shown by rotor angles that swing together and remain within 180 degrees of each other in the first second or so following a disturbance. Transient stability overall is indicated by the damping of oscillations and the system’s ability to maintain synchronous operation for the first 2-3 seconds following a disturbance. Figures 7-3 and 7-4 both demonstrate stable response to severe contingencies, three-phase faults at Ghorasal 230 kV and Meghnaghat 230 kV, respectively.
Rotor angle plots for all contingencies are included in Appendix B.
Figure 7-3 Stability Plot of NCF-01
Figure 7-4 Stability Plot of NCF-04
7.5.5 System Components Added
The 230 KV and above transmission system components considered by the study are listed in the following tables. The transmission lines and transformers for Plan A are shown in Tables 7-8 and 7-9, respectively.
Table 7-8 Summary of Transmission Lines for 2025 Transmission Plan A
Year | Bus # | Name | Bus # | Name | # Added CKT | KM |
2025 | 2006 | MAWA 230 | 2034 | AMINBAZA 230 | 1 | 40.00 |
2025 | 2012 | HARIPUR 230 | 2014 | MEGHNAGH 230 | 1 | 11.60 |
2025 | 2034 | AMINBAZA 230 | 2045 | OLDRPT 230 | 1 | 10.00 |
2025 | 2009 | SIKALBAHA 230 | 2026 | MAND/SIKALB 230 | 2 | 20.00 |
2025 | 2018 | MADANGANJ 230 | 2019 | SITALAKHYA 230 | 2 | 4.50 |
2025 | 2051 | BHANDARIA 230 | 2053 | BARISAL 230 | 2 | 40.00 |
2025 | 2006 | MAWA 230 | 2013 | HASNABAD 230 | 3 | 30.00 |
2020 | 2002 | HATHAZARI 230 | 2007 | MANDAHAT 230 | 1 | 9.00 |
2020 | 2011 | TONGI 230 | 2022 | UTTARA 230 | 1 | 9.00 |
2020 | 2012 | HARIPUR 230 | 2014 | MEGHNAGH 230 | 1 | 11.60 |
2020 | 2023 | MIRPUR 230 | 2034 | AMINBAZA 230 | 1 | 10.00 |
2020 | 2002 | HATHAZARI 230 | 2025 | BARAULIA 230 | 2 | 12.00 |
Year | Bus # | Name | Bus # | Name | # Added CKT | KM |
2020 | 2006 | MAWA 230 | 2013 | HASNABAD 230 | 2 | 30.00 |
2020 | 2006 | MAWA 230 | 2034 | AMINBAZA 230 | 2 | 40.00 |
2020 | 2007 | MANDAHAT 230 | 2024 | KULSHI 230 | 2 | 12.70 |
2020 | 2013 | HASNABAD 230 | 2019 | SITALAKHYA 230 | 2 | 12.00 |
2020 | 2022 | UTTARA 230 | 2023 | MIRPUR 230 | 2 | 13.00 |
2020 | 2024 | KULSHI 230 | 2025 | BARAULIA 230 | 2 | 12.90 |
2020 | 2007 | MANDAHAT 230 | 2026 | MAND/SIKALB 230 | 3 | 30.00 |
2015 | 2014 | MEGHNAGT 230 | 2029 | SHAMPUR TAP 230 | 1 | 16.00 |
2015 | 2002 | HATHAZARI 230 | 2007 | MANDAHAT 230 | 2 | 9.00 |
2015 | 2007 | MANDAHAT 230 | 2026 | MAND/SIKALB 230 | 2 | 30.00 |
2015 | 2009 | SIKALBAHA 230 | 2026 | MAND/SIKALB 230 | 2 | 20.00 |
2015 | 2023 | MIRPUR 230 | 2034 | AMINBAZAR 230 | 2 | 10.00 |
2010 | 2003 | MANIKNAGR 230 | 2004 | SIDDHIRGANJ 230 | 2 | 11.00 |
2010 | 2011 | TONGI 230 | 2039 | KASIMPUR 230 | 2 | 15.00 |
2010 | 2039 | KASIMPUR 230 | 2050 | KABIRPUR 230 | 2 | 11.00 |
2010 | 2034 | AMINBAZA 230 | 2045 | OLDRPT 230 | 2 | 10.00 |
2010 | 2014 | MEGHNAGT 230 | 2034 | AMINBAZAR 230 | 2 | 48.00 |
Table 7-9 Summary of Transformers for 2025 Transmission Plan A
Year | Bus# | Bus Name | Bus# | Bus Name | Units | MVA |
2025 | 1005 | MADANHAT 132 | 2007 | MADANHAT 230 | 2 | 225/225.0 |
2025 | 1006 | SIKALBAHA 132 | 2009 | SIKALBAHA 230 | 2 | 225/225.0 |
2025 | 1030 | COMILLA-N 132 | 2005 | COMILLA-N 230 | 2 | 225/225.0 |
2025 | 1101 | HARIPUR 132 | 2012 | HARIPUR 230 | 1 | 225/225.0 |
2025 | 1107 | RAMPURA 132 | 2016 | RAMPURA 230 | 2 | 225/225.0 |
2025 | 1112 | MADANGANJ 132 | 2018 | MADANGANJ 230 | 3 | 225/225.0 |
2025 | 1113 | HASNABAD 132 | 2013 | HASNABAD 230 | 1 | 225/225.0 |
2025 | 1120 | MIRPUR 132 | 2023 | MIRPUR 230 | 1 | 225/225.0 |
2025 | 1125 | TONGI 132 | 2011 | TONGI 230 | 2 | 225/225.0 |
2025 | 1130 | GHORASAL 132 | 2010 | GHORASAR 230 | 3 | 125/125.0 |
2025 | 1134 | AMINBAZA 132 | 2034 | AMINBAZA 230 | 1 | 225/225.0 |
2025 | 1145 | OLDARPT 132 | 2045 | OLDARPT 230 | 3 | 225/225.0 |
2025 | 1146 | MODHUPUR 132 | 2052 | MODHUPUR 230 | 1 | 225/225.0 |
2025 | 1201 | ASHUGANJ 132 | 2008 | ASHUGANJ 230 | 2 | 225/225.0 |
2025 | 1320 | BARISAL 132 | 2053 | BARISAL 230 | 3 | 225/225.0 |
2025 | 1323 | BHANDARIA 132 | 2051 | BHANDARIA 230 | 1 | 225/225.0 |
2025 | 1332 | KHULNANW 132 | 2032 | KHUL23 230 | 4 | 225/225.0 |
2025 | 1442 | BARAPUKULIA 132 | 2042 | BARAPUKULIA 230 | 1 | 225/225.0 |
2020 | 1006 | SIKALBAH 132 | 2009 | SIKAL23 230 | 1 | 225/225.0 |
2020 | 1013 | KULSHI 132 | 2024 | KULSHI 230 | 3 | 225/225.0 |
2020 | 1015 | BARAULIA 132 | 2025 | BARAULIA 230 | 3 | 225/225.0 |
2020 | 1101 | HARIPUR 132 | 2012 | HARIPUR 230 | 1 | 225/225.0 |
2020 | 1123 | UTTARA 132 | 2022 | UTTARA 230 | 3 | 225/225.0 |
2020 | 1126 | KABIRPUR 132 | 2050 | KABRPR23 230 | 1 | 225/225.0 |
Year | Bus# | Bus Name | Bus# | Bus Name | Units | MVA |
2020 | 1134 | AMINBAZAR 132 | 2034 | AMINBAZAR 230 | 1 | 225/225.0 |
2020 | 1307 | JHENIDA 132 | 2049 | JHENIDA 230 | 2 | 225/225.0 |
2020 | 1440 | BOGRANEW 132 | 2040 | BOGRA 230 | 1 | 225/225.0 |
2020 | 2014 | MEGHNAGHAT 230 | 4014 | MEGHNAGHAT 400 | 2 | 375/375.0 |
2020 | 2034 | AMINBAZAR 230 | 4034 | AMINBAZAR 400 | 2 | 375/375.0 |
2015 | 1006 | SIKALBAHA 132 | 2009 | SIKALBAHA 230 | 1 | 225/225.0 |
2015 | 1030 | COMILLAN 132 | 2005 | COMILLAN 230 | 1 | 225/225.0 |
2015 | 1101 | HARIPUR 132 | 2012 | HARIPUR 230 | 2 | 225/225.0 |
2015 | 1104 | MANIKNAGAR 132 | 2003 | MANIKNAGAR 230 | 1 | 225/225.0 |
2015 | 1107 | RAMPURA 132 | 2016 | RAMPURA 230 | 1 | 225/225.0 |
2015 | 1113 | HASNABAD 132 | 2013 | HASNABAD 230 | 1 | 225/225.0 |
2015 | 1120 | MIRPUR 132 | 2023 | MIRPUR 230 | 4 | 225/225.0 |
2015 | 1129 | SHAMPTAP 132 | 2029 | SHAMPTAP 230 | 1 | 225/225.0 |
2015 | 1201 | ASHUGANJ 132 | 2008 | ASHUGANJ 230 | 1 | 225/225.0 |
2015 | 1332 | KHULNANW 132 | 2032 | KHUL23 230 | 1 | 225/225.0 |
2015 | 2014 | MEGHNAGHAT 230 | 4014 | MEGHNAGHAT 230 | 2 | 375/375.0 |
2015 | 2034 | AMINBAZAR 230 | 4034 | AMINBAZAR 400 | 2 | 375/375.0 |
2010 | 1006 | SIKALBAHA 132 | 2009 | SIKALBAHA 230 | 4 | 225/225.0 |
2010 | 1104 | MANIKNAGAR 132 | 2003 | MANIKNAGAR 230 | 2 | 225/225.0 |
2010 | 1113 | HASNABAD 132 | 2013 | HASNABAD 230 | 1 | 225/225.0 |
2010 | 1126 | KABIRPUR 132 | 2050 | KABIRPUR 230 | 3 | 225/225.0 |
2010 | 1139 | KASIMPUR 132 | 2039 | KASIMPUR 132 | 2 | 225/225.0 |
2010 | 1129 | SHAMPUR TAP 132 | 2029 | SHAMPUR TAP 230 | 3 | 225/225.0 |
2010 | 1134 | AMINBAZAR 132 | 2034 | AMINBAZAR 230 | 1 | 225/225.0 |
2010 | 1145 | OLDRPT 132 | 2045 | OLDRPT 230 | 3 | 225/225.0 |
2010 | 1307 | JHENIDA 132 | 2049 | JHENIDA 230 | 2 | 225/225.0 |
2010 | 1442 | BARAPUKU 132 | 2042 | BARAPUKU 230 | 1 | 150/150.0 |
Plan A includes all elements of Plan B with exception of the 400 KV transmission facilities that link the proposed Mawa generation center, located southwest of Dhaka to Dhaka’s Hasnabad 400 KV and Aminbazar 400 KV substations. The 400 KV components of Plan B are summarized in Table 7-10. The 400 kV transmission lines replace similar 230 kV facilities in Plan A.
As noted in Section 5.3, the options for transmission development are based on broad stroke assessment of the regional transfer requirements. Based on the generation and load forecast used in the transmission expansion plan development, it is our judgment that all transfer requirements can be carried out most effectively by using either 230 kV or 132 kV transmission options. Plan A, shown in Table 7-9, presents the 400, 230 and 132 kV option. Plan B, presented in Table 7-10, is similar to Plan A, except for the transmission from Mawa to Dhaka, which is planned for 400 kV.
Table 7-10 Summary of 2025 Transmission Plan B
Year | BUS# | NAME | BSKV | BUS# | NAME | BSKV | CKT | km |
2025 2025 | 4001 4001 | MAWA MAWA | 400 400 | 4002 4003 | AMINBAZ HASNAB | 400* 400* | 1 1 | 40 30 |
2020 2020 | 4001 4001 | MAWA MAWA | 400 400 | 4002 4003 | AMINBAZ HASNAB | 400* 400* | 1 1 | 40 30 |
Year | BUS# | NAME | BSKV | BUS# | NAME BS | KV | Units | MVA |
2025 2025 | 2013 2034 | HASN23 AMIN23 | 230 230 | 4003 4002 | HASNAB AMINBAZ | 400 400 | 3 3 | 375/375 375/375 |
2020 2020 | 2013 2034 | HASN23 AMIN23 | 230 230 | 4003 4002 | HASNAB AMINBAZ | 400 400 | 2 2 | 375/375 375/375 |
Table 7-11 shows the size and locations of capacitors larger than 100 MVAR by region needed in the system over the period 2005 - 2025. This is the same for Plan A and Plan B. Including smaller installations, about 4,715 MVAR of capacitors is needed by 2025. A table including all the transmission components identified in the horizon year is included in Appendix B
Table 7-11 Capacitors 100 MVARS and Larger for Horizon Year 2025
Bus Number | Bus Name | KV Level | Capacitor (MVAR) | Region |
1009 | COXBZR | 132 | 135 | Southern |
1021 | CHOWMW | 132 | 180 | Southern |
1031 | COMI-S | 132 | 135 | Southern |
2002 | HATHAZARI | 230 | 450 | Southern |
1104 | MANIKNAGAR | 132 | 135 | Dhaka |
1126 | KABIRPUR | 132 | 450 | Dhaka |
1127 | MANIKGANJ | 132 | 135 | Dhaka |
1128 | TANGAL | 132 | 180 | Dhaka |
1146 | MODHUPR | 132 | 135 | Dhaka |
2011 | TONGI | 230 | 180 | Dhaka |
1204 | JAMLPR | 132 | 135 | Central |
1306 | JESSORE | 132 | 270 | Western |
1313 | FARIDPUR | 132 | 135 | Western |
1432 | THAKURGAON | 132 | 135 | Northern |
2040 | BOGRA | 230 | 135 | Northern |
7.5.6 Economic Comparison of Plans
The economic comparison of the two transmission plans, i.e., Plan A and Plan B was conducted taking into consideration the cost of the main components of the plans. The components considered in the economic evaluation were 132 KV, 230 KV and 400 KV transmission lines, 400/230 KV and 230/132 KV transformers, switched capacitors, and breakers. The substation configuration considered as typical was breaker and a half, which
requires three circuit breakers for every two terminations. Additionally the cost of transmission losses and operations and maintenance were included as part of the economic evaluations.
From 2005-2025, 104 new 132/33 kV substations and connecting 132 kV lines are needed, capable of serving demand of 12,480 MW. The corresponding cost of BDT 54.1 million is incorporated in the costs shown in Table 7-12. These costs were the same for Plans A and B. We emphasize that these results were not the product of load flow or other technical analysis. We simply compared the demand at each substation to its capacity, and added a new substation when the demand exceeded the capacity.
The present worth approach was used to refer the yearly cost of both plans to a common reference point in time, which for the Master Plan was 2005. We assumed that the investment costs for the components required in 2010 were incurred equally in each year 2007-2010. For years 2015, 2020, and 2025 we assumed that the investment costs in the study year were spread equally over that year and the preceding four years. The present worth cost of the Plan A is BDT 37,776 million and that of Plan B is BDT 38,383 million. In the economic analysis no escalation rate was used. The discount rate used is 12%. Table 7-12 shows a summary cost comparison for plans A and B. The cost tables that provide more detailed information of the cost analysis are included in Appendix B.
The Plan A net present value is BDT 607 million (equivalent to about $10 million) less than the Plan B net present value, a difference of about 2%.
Table 7-12 Summary Cost Comparison for Plan A and B
| TOTAL COST, BDT MILLIONS |
Year | Plan A | Plan B |
2005 | 351 | 351 |
2006 | 421 | 421 |
2007 | 4,426 | 4,426 |
2008 | 4,496 | 4,496 |
2009 | 4,566 | 4,566 |
2010 | 4,636 | 4,636 |
2011 | 5,771 | 5,771 |
2012 | 5,857 | 5,857 |
2013 | 5,943 | 5,943 |
2014 | 6,029 | 6,029 |
2015 | 6,115 | 6,115 |
2016 | 7,822 | 8,184 |
2017 | 8,005 | 8,367 |
2018 | 8,189 | 8,550 |
2019 | 8,372 | 8,733 |
2020 | 8,555 | 8,916 |
2021 | 11,406 | 11,842 |
2022 | 11,610 | 12,022 |
2023 | 11,813 | 12,202 |
2024 | 12,016 | 12,382 |
2025 | 12,220 | 12,562 |
Total | 148,618 | 152,370 |
| | |
Present Worth | 37,776 | 38,383 |
7.5.7 Cost of System Components Added
The unit cost of system components used for the cost analysis of the two plans was provided by PGCB. Where cost figures of components were not available we used typical costs data from similar options applied in other countries worldwide. To perform a cost analysis for the Master Plan the cost of transmission lines, transformers breakers and manually switched capacitors was taken into account. The cost of transmission lines, transformers and breakers needed between 2020 and 2025 to keep the transmission system within reliability criteria is shown in Table 7-13 for Plan A.
Table 7-13’s yearly values are somewhat smaller than the yearly values for Plan A shown in Table 7-12 because the Table 7-12 values include the costs of losses and O&M on the entire system, not just the components added in 2021- 2025.
From 2021-2025, 43 new 132/33 kV substations and connecting 132 kV lines are needed, capable of serving demand of 5,160 MW. The corresponding cost of BDT 22.4 million is incorporated in the costs shown in Table 7-13.
Table 7-13 Cost of Components for 2021-2025
Year | Cost, Million Taka |
2021 | BDT 9,020.6 |
2022 | BDT 9,020.6 |
2023 | BDT 9,020.6 |
2024 | BDT 9,020.6 |
2025 | BDT 9,020.6 |
7.5.8 Plan A vs. Plan B
In raw figures, Plan A is economically more attractive than Plan B, with a present worth difference of about 2%. Considering the level of total investment required for both plans, this difference is not major from a planning perspective.
Technically, higher voltage transmission facilities can handle larger power flows and at the same time have lower unit cost, less losses and less right-of-way requirements. However, Bangladesh is not a large geographic area, is not interconnected with other countries, has and will have substantial generation located close to the Dhaka load center, and thus will not be moving large blocks of power around the country. These offsetting factors make the analysis of a move to 400 KV a worthy topic for this study.
Based on the previous statements the approach during the transmission section of the Master Plan was to continue using 230 KV as the voltage level for new transmission facilities needed to continue the expansion of the transmission system in an economical and reliable fashion.
The proximity to the load centers of the proposed generation centers was a major factor for the 230 KV level to offer a viable solution to the expansion of the transmission system since there is not a need to transfer large blocks of power across the country.
The proposed level of generation of 2,800 MW at Mawa, located about 40 KM southwest of Dhaka, could be transferred using either 230 KV or 400 KV transmission facilities. Plan A
considered 230 KV as the voltage level to transfer power from Mawa to Dhaka City while Plan B considered 400 KV as the voltage level to accomplish the transfer of power to Dhaka.
As indicated above there is a short distance from Mawa to the Dhaka area so that 230 kV would be appropriate to transfer power from this new generation center to the Dhaka area. In addition to this Plan A has a lower cost figure than Plan B. However, a decision on this point is not needed now, because the first differences between the Plans do not appear until the 2020 study year.
7.5.9 Sensitivity Analysis
Sensitivity analysis was conducted for years 2025 and 2020 by modifying the base case dispatch. The sensitivity analysis was conducted to test the capability of the transmission plan to evacuate the system generation without violation of criteria. For 2025 two sensitivities were conducted as follows:
- In the Dhaka area existing generation at CDC IPP Haripur and CDC IPP Meghnaghat, which was off-line in the basic dispatch used to develop the transmission plan in 2025, was dispatched at full capability in place of generation in the proposed Mawa generation complex located southwest of Dhaka. Load flow analysis and contingency analysis were conducted indicating that the plan was within criteria. Results are shown in Appendix B.
- In the Southern Region existing generation at Rauzan, which was off-line in the basic dispatch used to develop the transmission plan in 2025, was dispatched at full capability in place of generation in the proposed Mawa generation complex located southwest of Dhaka. Load flow analysis and contingency analysis were conducted indicating that the plan was within criteria. Results are shown in Appendix B.
7.5.10 Recommendations
A transmission plan is in constant need of revision to take into consideration changes in assumptions that are needed to study the future development of the transmission system. The review of previous Master Plans has taken place every ten years. In a period of ten years it is very likely that the assumptions used in the previous plan could change considerably. A shorter period of time, for example, five years or even more frequently could be more appropriate for reviewing these plans.
In preparation for PGCB’s future transmission studies, details on the feasibility of certain routes should be determined. This may be of more benefit for the Dhaka area where obtaining right of way could prove more difficult than in the other regions of the country.
Development of a dynamic data base of the existing generating facilities is of great importance to evaluate the dynamic response of the transmission system. This will require a coordinated effort between the owners of generating facilities and PGCB.
7.5.11 Problems/Issues
During the transmission study we identified serious voltage problems the 132 KV system in the Central region, with all transmission facilities in service. We found out that dispatching
the Mymesing generating unit solved the voltage problem. Based on this we concluded that the generation at Mymesing is a “Must Run” generating facility.
7.6 2010 PLAN
The transmission systems for 2010, 2015, and 2020 are the result of back staging the transmission plan developed in the horizon year. As indicated in the previous section we have chosen Plan A for this study. Based on this we have chosen Plan A as the transmission plan for the back staging process.
Staging is the process of determining how the horizon year plan can be effectively developed from the base year transmission configuration. The objective is to determine when the components of the transmission plan are needed to meet criteria. The 2010 load flow base case obtained from the back staging process was tested using load flow and contingency analysis to verify that it is in compliance with reliability criteria. The 2010 substation peak load forecast and the corresponding generation dispatch based on dispatch principles described in Section 7-4 were used for the development of the 2010 load flow base case.
Figure 7-5 shows the 400 kV (committed by PGCB, insulated for 400 kV but initially operated at 230 kV) and 230 KV components of the transmission plan by study year 2010. The load flow data base used for load flow and contingency analysis in 2010 is included in Appendix B.
Figure 7-5 One-Line Diagram of Plan A for Year 2010 (On following page)
-11.33
-22.23
1440 BOGNW
1.014
-5.0
83.81
7.92
2042 BRPUK23
1.049
-2.6
11.35
-1.89
11.35
-1.89
230.00 25.85
69.10
2.40
102.50 %
69.10
2.40
102.50 %
1442 BARPUKR
1.024
-6.3
2052 SRIPR23
1.030
-1.4
57.15
34.99
1146 SRIPR132
1.016
-2.8
-11.33
-22.23
-73.53
-15.58
-73.53
102.50 %
85.90
67.72
100.00 %
69.10
2.40
102.50 %
62.28
-16.36
-0.91
-53.06
-0.91
-53.06
100.00 %
57.15
34.99
100.00 %
-15.58
2040 BOG23
1.043
-21.92
62.28
-21.92
-7.55
-16.36
-7.55
56.41
-56.24
-42.05
-9.63
-42.05
-9.63
56.30
-25.31
56.30
-25.31
-2.9
73.80
1.76
73.80
1.76
2036 SRJGNJ
1.050
-1.5
1.03
35.16
1.03
35.16
1139 KASIM
0.999
-3.8
50.40
40.09
98.75 %
50.40
40.09
2039 KASIM23
1.004
-2.5
-125.45
37.79
125.65
-39.56
96.64
66.57
157.00
95.03
56.91
19.04
100.00 %
56.91
19.04
100.00 %
1130 GHRASL
1.011
-30.26
56.41
-30.26
42.20
-1.98
42.20
18.07
-56.24
18.07
112.09
1.80
100.00 %
112.09
150.17
2.48
150.17
2.48
-149.52
6.68
450.00
98.98
1412 BAGBRI
1.028
98.75 %
75.05
-77.88
-125.45
37.79
125.65
-39.56
157.00
95.03
157.00
95.03
127.43
56.91
19.04
100.00 %
56.91
19.04
-2.6
16.38
0.99
16.38
0.99
-1.98
96.51
13.58
100.00 %
1201 ASHUGJ
1.017
-5.0
1030 COMI-N
1.020
-4.4
1.80
100.00 %
-126.34
33.14
-7.21
-48.02
103.75 %
-3.4
49.97
-35.58
75.05
-77.88
-89.74
43.24
-126.55
-75.41
75.73
100.00 %
-3.35
61.53
423.00
4.66
-126.34
-149.52
-7.21
6.68
103.75 %
-89.74
43.24
-126.55
-75.41
127.43
75.73
-3.35
61.53
96.51
13.58
100.00 %
33.14
2005 COMI23
142.83
55.23
142.83
55.23
-141.86
-60.90
-141.86
-60.90
-48.02
103.75 %
2030 BAGHA230
1.044
-3.2
-61.27
-0.56
-61.27
-0.56
-74.95
76.23
-74.95
76.23
2050 KABIR23
1.008
-2.8
49.97
-35.58
103.75 %
49.97
-35.58
103.75 %
60.43
47.82
97.50 %
60.43
47.82
97.50 %
60.43
47.82
97.50 %
2011 TONG23
2010 GRSL23
1.023
-0.6
2003 MANKNG23
1.005
0.5
-82.10
-47.93
82.10
47.94
97.50 %
82.10
47.94
2008 ASHU23
1.025
-0.3
1104 MANKNG
1.011
-1.5
3.50
-70.44
52.86
6.52
100.83 %
52.86
6.52
100.83 %
52.86
6.52
1.019
-1.7
-55.77
4.93
-55.77
4.93
1125 TONGI
1.002
82.21
-82.10
97.50 %
3.50
100.83 %
2020 ISHRDI
1.021
-5.4
50.89
-9.83
100.00 %
1401 ISHRDI
1.025
1.007
-3.4
2045 OLD230
-1.9
48.37
82.21
-47.93
100.90
-70.44
52.86
-49.94
126.78
76.20
50.89
-6.6
2034 AMIN230
0.994
-1.4
61.81
48.37
1145 OLDAIR
394.00
51.88
97.50 %
6.52
100.83 %
-17.96
-49.94
126.78
76.20
2044 BMARA23
1.016
-9.83
100.00 %
50.89
-9.83
100.00 %
89.94
-47.12
89.94
-47.12
-302.15
-100.95
0.998
-1.1
100.74
-92.71
-47.70
-92.71
-47.70
1134 AMINBAZ
1.012
31.80
96.25 %
61.81
31.80
96.25 %
61.81
31.80
1.020
-2.9
2004 SIDGJ230
1.011
0.9
114.79
-25.22
114.79
-25.22
79.07
2012 HARI23
1.011
-114.770.8
25.01
-114.77
100.90
51.88
97.50 %
100.90
51.88
97.50 %
-154.85
-7.38
-154.85
-7.38
1003 HATHAZ
1.014
-7.9
-17.96
2002 HATZ23
1.027
-5.3
-5.7
-126.61
-77.23
-126.61
52.16
72.60
95.00 %
1310 BMARA
1.033
-7.2
-302.15
-100.95
-31.70
59.32
96.25 %
100.74
59.32
-3.6
96.25 %
1101 HARIPR
1.014
81.30
-5.14
25.01
1107 RAMPRA
1.011
-2.9
2016 RAMP23
1.006
-0.4
50.00
13.43
-77.23
74.45
4.65
74.45
4.65
52.16
72.60
95.00 %
12.88
-31.70
12.88
96.25 %
100.74
59.32
96.25 %
73.82
1113 HASNBD
1.014
-1.2
100.00 %
81.30
-5.14
100.00 %
81.30
-5.14
155.34
7.68
155.34
7.68
-179.98
21.58
-179.98
360.00
14.74
100.00
30.80
2001 RAJN23
1.030
-5.0
50.00
13.43
2032 KHUL23
1.002
-7.6
65.26
18.43
97.50 %
1332 KHULNW
1.020
-9.2
31.72
-17.32
19.41
97.50 %
73.82
19.41
-2.7
2029 SHMPR23
0.998
-0.4
78.11
82.14
93.48 %
100.00 %
1129 SHAMPR
21.58
2015 HAR360
1.011
127.15
-40.64
112.50
39.59
93.75 %
-74.12
-18.56
-74.12
-18.56
197.00
0.38
6.16
-1.85
6.16
-1.85
67.95
20.42
98.75 %
-6.15
-18.31
-6.15
-18.31
65.26
18.43
97.50 %
39.38
-4.26
39.38
-4.26
39.33
3.82
97.50 %
39.33
3.82
97.50 %
31.72
-17.32
2013 HASN23
0.996
-0.9
97.50 %
73.82
19.41
97.50 %
-142.45
-11.78
-142.45
-11.78
142.62
10.81
142.62
10.81
78.11
82.14
93.48 %
78.11
82.14
93.48 %
1.034
-2.3
-259.78
-134.01
-259.78
-134.01
17.44
-46.57
17.44
-46.57
0.9
-17.42
44.21
-17.42
44.21
260.87
138.10
260.87
138.10
127.15
-40.64
450.00
217.04
450.00
260.04
450.00
1006 SIKLBHA
1.019
-7.8
112.50
39.59
93.75 %
112.50
39.59
93.75 %
112.50
450.00
190.73
67.95
20.42
2051 BAGHER23
304.40
49.56
1.14
39.59
93.75 %
2049 JHNDHA23
1.007
-7.4
98.75 % 1307 JHNDHA 1.009
-9.4
1.001
-8.1
1323 BAGHER132
1.025
-9.0
304.40
49.56
2014 MEGH23
1.013
0.7
2009 SIKBHA23
0.973
-4.5
7.6.1 Load Flow and Contingency Analysis Results
A number of transmission facilities from Plan A were identified for implementation in the period 2005-2010 in order to maintain the transmission system operating within reliability criteria. Following is a description of those facilities:
- In the Dhaka area we identified the following transmission facilities that need to be considered during the implementation stage for the period 2005-2010:
− Two new circuits from Aminbazar 230 KV to Old Airport 230 KV intended to transfer power to a group of proposed substations which purpose will be to relieve the loading of existing substations before these become overloaded. Old Airport 230/132 KV is a proposed substation.
− Two new circuits from Old Airport 132 KV to Dhaka University 132 KV. Dhaka University is a proposed substation intended to relieve the loading of Kollyanpur 132 KV and Dhanmondi 132 KV substations.
− Two new circuits from Old Airport 132 KV to Cantonment 132 KV. Cantonment is a proposed substation intended to relieve the loading of the Mirpur substation.
− Two new circuits from Aminbazar 132 KV to Savar 132 KV. Savar is a proposed substation intended to relieve the loading of Mirpur, Kollyanpur and Kabirpur substations.
− Two new circuits from Joydevpur 132 KV to Modhupur 132 KV. Modhupur is a proposed substation intended to relieve the loading of the Joydevpur 132 KV substation.
− A new tap called Kasimpur on the two circuits from Tongi 132 KV to Kabirpur 132 KV. This proposed tap will is intended to relieve the loading of the Joydevpur 132 KV substation.
− A new tap called Shampur on the two circuits from Meghnaghat 230 kV substation to Hasnabad 230 kV substation.
− Two new circuits from Tongi 230 to a new Kasimpur 230 kV and another two circuits from the new Kasimpur 230 kV substation to a new Kabirpur 230 kV substation. These circuits will assist in relieving overloads under contingency conditions after the loss of several single parallel circuits in the northeast portion of Dhaka.
− Two new circuits from Meghnaghat to Aminbazar. These circuits have being committed by PGCB and will be insulated at 400 kV though initial operation in 2010 will be at 230 kV.
− A new tap called Shrimpur on the two circuits from Ashuganj 230 kV substation to Sirajganj 230 kV substation.
- In the Southern region we identified, after discussion with PGCB planning staff, the following transmission facility which needs to be considered during the implementation stage for the period 2005-2010 :
− A short 132 KV circuit to connect the existing Baraulia 132 KV to Comilla North 132 KV line to Feni 132 KV substation. The section of the line from Feni to Comilla North will be dismantled and used to build a new circuit from Natore 132 KV to Rajshahi 132 KV substations.
- In the Western region we identified the following transmission facility which needs to be considered during the implementation stage for the period 2005-2010:
− Two new circuits from Bhola 132 KV to Barisal 132 KV. These circuits should come into service when the planned generation at Bhola comes into service.
− Two new circuits from Khulna 230 kV to a new Bhandaria 230 kV substation. These circuits will provide a higher level of reliability to this region and will contribute to eliminate overloads and low voltage problems under N-1 conditions.
− Several 132 kV circuits like Jhenida 132 kV to Chuadanga 132 kV and Jhenida 132 kV to Magura 132 kV. These projects were provided as committed projects by PGCB.
- In the Northern region we identified the following transmission facility which needs to be considered during the implementation stage for the period 2005-2010:
− A new 132 KV circuit between Natore 132 KV to Rajshahi 132 KV substations. This circuit is intended to be built using the conductor from the section of the line from Feni to Comilla North once it is dismantled.
− Several 132 kV circuits like Thakurgaon 132 kV to Panchagar 132 kV and Joypurhat 132 kV to Naogaon 132 kV. These projects were provided as committed projects by PGCB.
Transformers needed in place by 2010 are listed below:
- One 230/132 KV of 225/225 MVA at Aminbazar substation
- One 230/132 KV of 225/225 MVA at Hasnabad substation
- Three 230/132 KV of 225/225 MVA at Kabirpur substation
- Two 230/132 KV of 225/225 MVA at Kasimpur substation
- One 230/132 KV of 150/150 MVA at Barapukuria substation
- Three 230/132 KV of 225/225 MVA at Old Airport substation
- Two 230/132 KV of 225/225 MVA at Jhenida substation
- Four 230/132 KV of 225/225 MVA at Sikalbaha substation
- Three 230/132 KV of 225/225 MVA at Shampur tap
- Two 230/132 KV of 225/225 MVA at Maniknagar substation
Reactive compensation for the period 2005-2010 by the application of shunt capacitors was considered in the Master Plan transmission study. The level of reactive compensation to keep voltages in the 230 KV and 132 KV systems within reliability criteria is 1340 MVAR. Appendix B lists the size and location of capacitors for the period 2005-2010.
As indicated in a previous section, the objective of identifying the location and size of shunt capacitors is to determine the level of reactive compensation needed to keep voltages within criteria and to prevent voltage collapse. The final location and size of the capacitors identified by this study could change depending on the location of new 132 KV and 230 KV substations that would supply portions of the load from existing substations to avoid becoming overloaded during the 2005 to 2010 period.
Table 7-14 shows the level of reactive compensation by region by the year 2010. The table shows that the level of reactive compensation in general tends to be higher in the major load centers in the country like Dhaka and the Chittagong area in the Southern region.
Table 7-14 Reactive Compensation by Region for 2010
Region | Reactive Compensation (MVAR) |
Southern | 315 |
Dhaka | 450 |
Central | 115 |
Western | 205 |
Northern | 255 |
Total | 1340 |
7.6.2 System Summary – Generation Load Losses
Table 7-15 shows active and reactive generation in MW and MVAR, respectively, active and reactive loads in MW and MVAR, respectively, reactive power from capacitors in MVAR, reactive power due to transmission lines capacitive effect in MVAR, and active and reactive losses by region. The total active losses are about 1.2% of total net load while reactive power losses are substantially higher.
Table 7-15 Generation Load and Losses by Region 2010
| GENERATION | LOAD | CAPAC- ITORS | LINE CHARGING | LOSSES |
REGION | MW | MVAR | MW | MVAR | (MVAR) | (MVAR) | MW | MVAR |
SOUTHERN | 900 | 456.2 | 1308.5 | 633.6 | 235.6 | 139.2 | 10.4 | 163.4 |
DHAKA | 3857.6 | 1220.6 | 3014 | 1459.4 | 266.9 | 388.3 | 25.9 | 578.4 |
CENTRAL | 283 | 168.3 | 550 | 266.3 | 119.4 | 51.9 | 8.4 | 58.9 |
WESTERN | 447 | 25.1 | 767.3 | 371.6 | 216.1 | 173.3 | 10.8 | 82.4 |
NORTHERN | 1001.0 | 180.9 | 773.1 | 374.1 | 262.9 | 260.5 | 20.2 | 177.2 |
TOTALS | 6488.6 | 2051.1 | 6412.9 | 3105 | 1100.9 | 1013.2 | 75.7 | 1060.3 |
7.6.3 System Components Added
The components of the transmission plan for the period 2005-2010 are shown in Tables 7-16, 7-17, and 7-18. Table 7-16 shows the list of transmission lines identified by the plan that need to be implemented by 2010.
Table 7-16 List of Transmission Lines for 2010
Year | Bus # | Name | Bus # | Name | # Added CKT | KM |
2010 | 1015 | BARAULIA 132 | 1020 | FENI 132 | 1 | 1.00 |
2010 | 1201 | ASHUGANJ 132 | 1202 | KISHORGANJ 132 | 1 | 51.80 |
2010 | 1403 | NATORE 132 | 1405 | RAJSHAHI 132 | 1 | 40.00 |
2010 | 1415 | BOGRA 132 | 1440 | BOBOGRANEW 132 | 1 | 2.00 |
2010 | 1125 | TONGI 132 | 1139 | KASIMPUR 132 | 2 | 15.00 |
2010 | 1126 | KABIRPUR 132 | 1139 | KASIMPUR 132 | 2 | 11.00 |
2010 | 1132 | JOYDEVPU 132 | 1146 | MODHUPUR 132 | 2 | 32.00 |
2010 | 1134 | AMINBAZA 132 | 1135 | SAVAR 132 | 2 | 10.00 |
2010 | 1142 | UNIVRSIT 132 | 1145 | OLDARPT 132 | 2 | 6.50 |
2010 | 1143 | CANTOMNT 132 | 1145 | OLDARPT 132 | 2 | 6.00 |
2010 | 1215 | SYLHET 132 | 1250 | SILNWPS 132 | 2 | 3.00 |
2010 | 1304 | CHUADANGA 132 | 1307 | JHENIDA 132 | 2 | 40.00 |
2010 | 1307 | JHENIDA 132 | 1309 | MAGURA 132 | 2 | 33.00 |
2010 | 1416 | JOYPURHAT 132 | 1417 | NAOGAON | 2 | 40.00 |
2010 | 1328 | BHOLA 132 | 1320 | BARISAL 132 | 2 | 55.00 |
2010 | 1431 | PANCHAGAR 132 | 1432 | THAKURGAON | 2 | 45.00 |
2010 | 1111 | SHAMPUR 132 | 1129 | SHAMPUR TAP 132 | 3 | 2.00 |
2010 | 2003 | MANIKNAGAR 230 | 2004 | SIDDHIRGANJ 230 | 2 | 11.00 |
2010 | 2011 | TONGI 230 | 2039 | KASIMPUR 230 | 2 | 15.00 |
2010 | 2039 | KASIMPUR 230 | 2050 | KABIRPUR 230 | 2 | 11.00 |
2010 | 2034 | AMINBAZA 230 | 2045 | OLDRPT 230 | 2 | 10.00 |
2010 | 2014 | MEGHNAGHAT 230 | 2034 | AMINBAZAR 230 | 2 | 48.00 |
Table 7-17 List of Transformers for 2010
Year | Bus # | Name | Bus # | Name | # Added Trnsfrmrs | MVA |
2010 | 1006 | SIKALBAHA 132 | 2009 | SIKALBAHA 230 | 4 | 225/225.0 |
2010 | 1104 | MANIKNAGAR 132 | 2003 | MANIKNAGAR 230 | 2 | 225/225.0 |
2010 | 1113 | HASNABAD 132 | 2013 | HASNABAD 230 | 1 | 225/225.0 |
2010 | 1126 | KABIRPUR 132 | 2050 | KABIRPUR 230 | 3 | 225/225.0 |
2010 | 1139 | KASIMPUR 132 | 2039 | KASIMPUR 132 | 2 | 225/225.0 |
2010 | 1129 | SHAMPUR TAP 132 | 2029 | SHAMPUR TAP 230 | 3 | 225/225.0 |
2010 | 1134 | AMINBAZAR 132 | 2034 | AMINBAZAR 230 | 1 | 225/225.0 |
2010 | 1145 | OLDRPT 132 | 2045 | OLDRPT 230 | 3 | 225/225.0 |
2010 | 1307 | JHENIDA 132 | 2049 | JHENIDA 230 | 2 | 225/225.0 |
2010 | 1442 | BARAPUKU 132 | 2042 | BARAPUKU 230 | 1 | 150/150.0 |
Table 7-18 shows the location and size of capacitors larger than 25 MVAR that need to be implemented by 2010. The table showing all capacitors for 2010 is included in Appendix B.
Table 7-18 Capacitors for 2010 Larger Than 25 MVAR
Bus Number | Bus Name | Voltage Level KV | Capacitors (MVAR) |
1003 | HATHAZRI | 132 | 45 |
1008 | DOHAZARI | 132 | 45 |
1009 | COXBZR | 132 | 45 |
1016 | BAKUL | 132 | 45 |
1020 | FENI | 132 | 45 |
1021 | CHOWMW | 132 | 50 |
1031 | COMI-S | 132 | 45 |
1032 | CHANDPR | 132 | 45 |
1103 | MOGBAZ | 132 | 45 |
1106 | DHANMO | 132 | 45 |
1107 | RAMPURA | 132 | 90 |
1128 | TANGAL | 132 | 45 |
1134 | AMINBAZAR | 132 | 90 |
2045 | OLDRPT | 230 | 90 |
2050 | KABIRPUR | 230 | 45 |
1202 | KISHGJ | 132 | 45 |
1205 | NETROK | 132 | 45 |
1306 | JESORE | 132 | 45 |
1313 | FRIDPR | 132 | 45 |
1315 | MADAPR | 132 | 45 |
1320 | BRISAL | 132 | 45 |
1403 | NATORE | 132 | 45 |
1406 | NWBGNJ | 132 | 45 |
1416 | JOYPHT | 132 | 45 |
1421 | LALMON | 132 | 45 |
1425 | SAIDPR | 132 | 50 |
7.6.4 Cost of System Components Added
This section shows the cost of the plan components for the period 2005-2010. The cost of system components used for the cost analysis was provided by PGCB. Where cost figures of components were not available, we used typical costs data from similar options applied in other countries worldwide. To perform a cost analysis for the Master Plan the cost of transmission lines, transformers, breakers, and manually switched capacitors was taken into account. The cost of transmission lines, transformers, and breakers needed between 2005 and 2010 to keep the transmission system within reliability criteria is shown in Table 7-19.
From 2006-2010, five new 132/33 kV substations and connecting 132 kV lines are needed, capable of serving demand of 600 MW. The corresponding cost of BDT 2.6 million is incorporated in the costs shown in Table 7-19.
Table 7-19 Cost of Components for 2005-2010
Year | Cost, Million Taka |
2007 | 3,877 |
2008 | 3,877 |
2009 | 3,877 |
2010 | 3,877 |
7.6.5 Recommendations
It is important to initiate the studies for the implementation stage of the transmission plan as soon as possible given the short period of time available to 2010.
7.7 2015 PLAN
As previously indicated the transmission systems for 2010, 2015, and 2020 are the result of back staging the Plan A transmission plan developed in the horizon year.
The 2015 load flow base case obtained from the back staging process was tested using load flow and contingency analysis to verify that it is in compliance with reliability criteria. The 2015 substation peak load forecast and the corresponding generation dispatch based on dispatch principles described in Section 7-4 were used for the development of the 2015 load flow base case.
Figure 7-6 shows the 400 kV and 230 KV components of the transmission plan by study year 2015. The load flow data base used for load flow and contingency analysis in 2015 is included in Appendix B.
Figure 7-6 One-Line Diagram of Plan A for Year 2015 (On following page)
1440 BOGNW
1.031
2042 BRPUK23
1.041
2.0
-29.22
-0.15
-29.22
-0.15
96.15
27.09
100.00 %
96.15
27.09
1442 BARPUKR
1.019
-3.0
2052 SRIPR23
1.020
1146 SRIPR132
29.30
-23.23
29.30
-23.23
-145.99
6.67
-145.99
0.2
116.69
16.55
100.00 %
116.69
16.55
100.00 %
230.00
106.57
100.00 %
96.15
27.09
100.00 %
-43.62
-66.53
300.00
1.9
-169.75
-23.97
-169.75
-23.97
115.58
25.20
100.00 %
115.58
25.20
100.00 %
1.011
-1.0
-23.02
-53.13
6.67
2040 BOG23
1.037
-12.82
-43.62
-12.82
-10.84
-66.53
-10.84
43.53
-54.04
-10.95
54.18
-1.23
-18.98
-23.02
-18.98
15.78
-53.13
15.78
3.0
147.07
-14.67
147.07
-14.67
131.34
5.00
131.34
2036 SRJGNJ
1.041
5.9
171.58
18.17
171.58
18.17
450.00
48.62
450.00
1139 KASIM
0.993
-4.5
53.16
-45.61
103.75 %
53.16
-45.61
103.75 %
2039 KASIM23
1.008
-3.1
-153.13
141.53
-153.13
141.53
153.65
-141.15
153.65
-141.15
-112.34
21.32
-112.34
56.42
191.73
107.00
45.90
107.00
45.90
107.00
45.90
63.47
48.80
96.67 %
63.47
48.80
96.67 %
63.47
48.80
96.67 %
1130 GHRASL
1.015
-2.6
66.82
5.82
66.82
-54.04
-10.95
23.08
7.03
23.08
7.03
76.11
6.42
100.00 %
54.18
-1.23
1201 ASHUGJ
1.013
-2.9
1030 COMI-N
1.001
108.23
14.89
100.00 %
108.23
14.89
100.00 %
108.23
14.89
100.00 %
5.00
-130.83
38.74
48.15
99.97
-95.92
99.97
60.71
29.68
21.32
-164.65
165.78
63.47
48.80
96.67 %
6.15
5.82
76.11
6.42
100.00 %
-2.6
-86.20
-19.14
-10.57
-130.83
-10.57
92.09
27.20
-16.64
101.25 %
38.74
-16.64
101.25 %
-99.81
94.68
66.54
-47.74
103.75 %
66.54
-47.74
-95.92
97.50 %
60.71
29.68
97.50 %
-20.18
-164.65
-20.18
21.86
165.78
21.86
2010 GRSL23
1.009
54.09
6.15
54.09
2008 ASHU23
1.016
76.11
6.42
100.00 %
61.64
17.11
-86.20
-19.14
2005 COMI23
1.007
0.2
92.09
27.20
1412 BAGBRI
-99.81
94.68
103.75 %
66.54
64.57
190.98
-0.3
86.83
0.9
97.50 %
53.72
-91.70
-36.77
-91.70
-36.77
2030 BAGHA230
1.035
4.4
44.12
-11.92
44.12
-11.92
1.029
3.5
2050 KABIR23
1.013
-3.5
-47.74
103.75 %
1125 TONGI
1.011
-3.7
91.67 %
60.71
29.68
97.50 %
77.90
28.72
2011 TONG23
0.998
-2.3
1120 MIRPU
1.007
-130.24
-76.84
-130.24
-76.84
51.24
97.50 %
86.83
51.24
97.50 %
86.83
117.89
66.18
96.25 %
117.89
66.18
-6.02
-62.95
61.64
17.11
97.50 %
61.64
17.11
-34.57
53.72
-34.57
2002 HATZ23
0.986
3.9
2020 ISHRDI
1.022
3.0
65.90
-8.49
100.00 %
1401 ISHRDI
1.025
2034 AMIN230
97.50 %
77.90
28.72
-3.6
2003 MANKNG
1.001
1.0
51.24
97.50 %
1104 MANKNG
1.005
-1.1
96.25 %
117.89
-6.02
-62.95
97.50 %
61.64
0.00
-51.28
61.42
-51.28
65.90
-8.49
1.5
0.997
-1.3 112.61
2023 MIRPU23
0.994
-1.7
97.50 %
77.90
130.54
66.18
96.25 %
-229.76
-69.42
-229.76
17.11
97.50 %
-4.48
0.00
-4.48
61.42 100.00 %
-24.75
116.97
-116.85 28.72
78.51
117.89
-69.42
-177.00
65.90
-8.49
450.00
112.61
-24.75
41.84
116.97
41.84
-43.07
-116.85
-43.07
97.50 %
130.54
78.51
66.18
96.25 %
1003 HATHAZ
0.998
0.8
4.82
-177.00
4.82
100.00 %
4034 AMIN400
102.23
-137.23
-28.81
91.49
48.87
394.00 85.55
2012 HARI23
1.009
1107 RAMPRA
2016 RAMP23
1005 MADNHT
2007 MADNHT23
0.987
2044 BMARA23
1.019
3.2
51.33
-63.28
80.39
83.38
95.00 %
1310 BMARA
1.031
0.9
1.015
1.7
-201.13
-148.14
201.13
148.16
98.13 %
107.15
60.01
96.25 %
-137.23
-28.81
96.25 %
91.49
48.87
96.25 %
400.00
86.05
266.47
-26.21
266.47
1.5
-266.38
26.47
230.96
1.006
-3.2
0.994
-0.3
450.00
200.96
1.000
1.2
109.29
53.44
96.25 %
4.4
80.39 -201.13 201.13
107.15
-266.38 74.68
450.00
51.33
-63.28
83.38
95.00 %
-148.14 148.16
98.13 %
60.01
96.25 %
2045 OLD230
0.994
91.49
48.87
96.25 %
2004 SIDGJ230
1.009
-26.21
97.96
13.61
26.47
230.96
74.68
200.96
109.29
53.44
96.25 %
177.23
-5.07
18.29
9.16
18.29
300.00
66.15
-101.53
-32.50
107.15
60.01
96.25 %
-1.8
1145 OLDAIR
1.013
1.6
100.00 %
97.96
13.61
-127.49
-50.91
-127.49
255.00
134.87
161.41
110.46
288.59
56.32
-286.52
-48.35
177.23
-5.07
9.16
98.04
20.93
1332 KHULNW
1.024
2.9
-101.53
-32.50
1134 AMINBAZ
1.011
-3.9
-4.0
100.00 %
97.96
13.61
-50.91
161.41 288.59
110.46 56.32
-286.52
-48.35
100.00 %
92.84
58.78
1113 HASNBD
1.014
100.00 %
2015 HAR360
1.010
2026 MD-SKL23
154.32
2001 RAJN23
0.987
-18.25
-25.20
450.00
139.55
197.00
-0.03
89.98
20.89
98.04
20.93
100.00 %
98.04
20.93
100.00 %
86.45
-1.65
86.09
10.36
100.00 %
101.77
30.32
101.77
30.32
95.83 %
92.84
58.78
95.83 %
92.84
58.78
95.83 %
92.84
58.78
95.83 %
-2.8 87.08
39.16
97.50 %
87.08
39.16
97.50 %
87.08
39.16
97.50 %
87.08
39.16
1101 HARIPR
1.004
-1.0
-308.23
-108.35
-308.23
-108.35
97.96
13.61
100.00 %
97.96
13.61
100.00 %
-81.99
-84.29
-81.99
-84.29
1.5
82.12
82.63
82.12
82.63
309.59
114.24
309.59
114.24
86.56
8.68
86.56
8.68
450.00
216.68
450.00
1.003
7.1
-160.79
-110.40
-160.79
-110.40
55.04
96.25 %
154.32
55.04
96.25 %
154.32
55.04
96.25 %
3.9
-0.00
-0.00
-0.00
-0.00
-18.25
-25.20
89.98
20.89
86.45
-1.65
86.09
10.36
2013 HASN23
0.995
-287.45
97.50 % -308.23
-108.35
288.17
23.55
309.59
114.24
259.70
154.32
55.04
107.59
62.56
97.50 %
2032 KHUL23
1.032
100.00 %
-0.6
-81.36
-287.45
84.21
288.17
2029 SHMPR23
1.002
450.00
-45.09
12.56
100.00 %
319.00
109.34
450.00
82.73
96.25 %
2049 JHNDHA23
1.010
2.8
107.59
62.56
97.50 %
5.3
1307 KHUL132
1.006
-0.3
2051 BAGHER23
1.026
3.6
1323 BAGHER132
1.022
1.5
-81.36
84.21
0.3
201.45
-43.62
201.45
-43.62
23.55
12.56
100.00 %
2014 MEGH23
1.015
1.7
2009 SIKBHA23
0.988
6.2
154.32
55.04
96.25 %
1006 SIKLBHA
1.001
1.6
7.7.1 Load Flow and Contingency Analysis Results
A number of transmission facilities from the horizon year plan were identified for implementation in the period 2010-2015 in order to maintain the transmission system operating within reliability criteria. Following is a description of those facilities:
- In the Dhaka area we identified the following 230 kV transmission facilities that need to be considered during the implementation stage for the period 2010-2015:
− The addition of one 230 KV circuit from Meghnaghat to Shampur tap. This additional circuit will assist in relieving overloads on the other two circuits between Meghnaghat and Shampur tap.
− The addition of two 230 kV circuits from Aminbazar to a new Mirpur 230 kV substation.
- In the Southern region we identified the following 230 kV transmission facilities that need to be considered during the implementation stage for the period 2010- 2015:
− Two 230 KV circuits from the Mandahat/New Sikalbaha generation site to Mandahat and Sikalbaha substations. The construction of these two circuits could be delayed if the construction of the proposed Mandahat/New Sikalbaha generation center is delayed. An expansion of the Mandahat substation will be necessary to accommodate 230 KV facilities.
− The construction of two 230 kV circuits from Hathazari to Mandahat
Reactive compensation for the period 2010-2015 by the application of shunt capacitors was considered by the Master Plan transmission study. The level of reactive compensation to keep voltages in the 230 KV and 132 KV systems within reliability criteria is 590 MVAR in addition to the 1340 MVAR needed in the period 2005 to 2010. A table listing the size and location of capacitors for the period 2010-2015 is included in Appendix B.
As indicated in a previous section the objective of identifying the location and size of shunt capacitors is to determine the level of reactive compensation needed to keep voltages within criteria and to prevent voltage collapse. The final location and size of the capacitors identified by this study could change depending on the location of new 132 KV and two 230 KV substations.
Table 7-20 shows the level of reactive compensation by region for the year 2015. The table shows that the level of reactive compensation in general tends to be higher in the major load centers in the country like Dhaka and the Chittagong area in the Southern region.
Table 7-20 Reactive Compensation by Region for 2015
Region | Reactive Compensation, (MVAR) |
Southern | 405 |
Dhaka | 900 |
Central | 115 |
Western | 300 |
Northern | 210 |
Region | Reactive Compensation, (MVAR) |
Total | 1,930 |
The MVAR shown in Table 7-20 includes the MVAR needed for the period 2005 to 2015. In other words, these are cumulative figures.
7.7.2 System Summary – Generation Load Losses
Table 7-21 shows active and reactive generation in MW and MVAR, respectively, active and reactive loads in MW and MVAR, respectively, reactive power from capacitors in MVAR, reactive power due to transmission lines capacitive effect in MVAR and active and reactive losses by region. The total active losses are about 1.3% of total net load while reactive power losses are substantially higher.
Table 7-21 Generation Load and Losses by Region 2015
| GENERATION | LOAD | CAPACITORS | LINE CHARGING | LOSSES |
REGION | MW | MVAR | MW | MVAR | (MVAR) | (MVAR) | MW | MVAR |
SOUTHERN | 1700 | 725.1 | 1938 | 938.4 | 321.8 | 164.7 | 21.8 | 349.3 |
DHAKA | 4569.2 | 1629 | 4459.2 | 2159.1 | 720.1 | 660.4 | 39.9 | 774.7 |
CENTRAL | 588 | 246.3 | 813.8 | 394.1 | 118.2 | 55.3 | 14 | 100.3 |
WESTERN | 1197 | 262.2 | 1136.8 | 550.6 | 253.5 | 184.8 | 23.4 | 201 |
NORTHERN | 1560 | 487.8 | 1144.4 | 553.9 | 209.7 | 261 | 22.9 | 278.5 |
TOTALS | 9614.2 | 3350.4 | 9492.2 | 4596.1 | 1622.3 | 1326.2 | 122 | 1703.8 |
7.7.3 System Components Added
The transmission lines of the transmission plan for the period 2010-2015 are shown in Table 7-22. Table 7-23 shows transformers for 230/132 KV substations and Table 7-24 shows capacitors larger than 100 MVAR. Tables listing all facilities are included in Appendix B.
Table 7-22 Transmission Lines for Period 2010 to 2015
Year | Bus # | Name | Bus # | Name | # Added Trnsfrmrs | KM |
2015 | 1006 | SIKALBAH 132 | 1008 | DOHAZARI 132 | 1 | 32.20 |
2015 | 1006 | SIKALBAH 132 | 1016 | BAKULIA 132 | 1 | 7.00 |
2015 | 1006 | SIKALBAH 132 | 1017 | JULDA 132 | 1 | 5.00 |
2015 | 1011 | HALISHAHAR 132 | 1013 | KULSHI 132 | 1 | 13.50 |
2015 | 1030 | COMILLAN 132 | 1031 | COMILLAS 132 | 1 | 15.90 |
2015 | 1118 | KAMRANGI 132 | 1122 | KALYANPU 132 | 1 | 12.00 |
2015 | 1124 | BASHUN 132 | 1144 | BHASUND 132 | 1 | 2.00 |
2015 | 1130 | GHORASAL 132 | 1131 | NARSHIND 132 | 1 | 16.00 |
2015 | 1101 | HARIPUR 132 | 1109 | MATUAIL 132 | 1 | 10.00 |
2015 | 1101 | HARIPUR 132 | 1136 | PURBCHL 132 | 1 | 25.00 |
2015 | 1103 | MOGHBAZAR 132 | 1107 | RAMPURA 132 | 1 | 4.50 |
2015 | 1105 | ULLON 132 | 1106 | DHANMOMDI 132 | 1 | 5.50 |
2015 | 1113 | HASNABAD 132 | 1118 | KAMRANGI 132 | 1 | 11.00 |
2015 | 1131 | NARSHIND 132 | 1136 | PURBCHL 132 | 1 | 13.00 |
2015 | 1134 | AMINBAZAR 132 | 1135 | SAVAR 132 | 1 | 10.00 |
Year | Bus # | Name | Bus # | Name | # Added Trnsfrmrs | KM |
2015 | 1202 | KISHORGANJ 132 | 1203 | MYMENSINGJ 132 | 1 | 58.90 |
2015 | 1302 | KHULNA C 132 | 1332 | KHULNANW 132 | 1 | 21.00 |
2015 | 1415 | BOGRA 132 | 1417 | NAOGOAN 132 | 1 | 45.00 |
2015 | 1425 | SAIDPUR 132 | 1430 | PURBSADIPUR 132 | 1 | 20.90 |
2015 | 1011 | HALISHAHAR 132 | 1017 | JULDA 132 | 2 | 8.00 |
2015 | 2014 | MEGHNAGHAT 230 | 2029 | SHAMPUR TAP 230 | 1 | 16.00 |
2015 | 2002 | HATHAZARI 230 | 2007 | MANDAHAT 230 | 2 | 9.00 |
2015 | 2007 | MANDAHAT 230 | 2026 | MAND/SIKALB 230 | 2 | 30.00 |
2015 | 2009 | SIKALBAHA 230 | 2026 | MAND/SIKALB 230 | 2 | 20.00 |
2015 | 2023 | MIRPUR 230 | 2034 | AMINBAZAR 230 | 2 | 10.00 |
Table 7-23 Transformers for Period 2010 to 2015
Year | Bus # | Name | Bus # | Name | # Added CKT | MVA |
2015 | 1006 | SIKALBAHA 132 | 2009 | SIKALBAHA 230 | 1 | 225/225.0 |
2015 | 1030 | COMILLAN 132 | 2005 | COMILLAN 230 | 1 | 225/225.0 |
2015 | 1101 | HARIPUR 132 | 2012 | HARIPUR 230 | 2 | 225/225.0 |
2015 | 1104 | MANIKNAGAR 132 | 2003 | MANIKNAGAR 230 | 1 | 225/225.0 |
2015 | 1107 | RAMPURA 132 | 2016 | RAMPURA 230 | 1 | 225/225.0 |
2015 | 1113 | HASNABAD 132 | 2013 | HASNABAD 230 | 1 | 225/225.0 |
2015 | 1120 | MIRPUR 132 | 2023 | MIRPUR 230 | 4 | 225/225.0 |
2015 | 1129 | SHAMPTAP 132 | 2029 | SHAMPTAP 230 | 1 | 225/225.0 |
2015 | 1201 | ASHUGANJ 132 | 2008 | ASHUGANJ 230 | 1 | 225/225.0 |
2015 | 1332 | KHULNANW 132 | 2032 | KHUL23 230 | 1 | 225/225.0 |
2015 | 2014 | MEGHNAGHAT 230 | 4014 | MEGHNAGHAT 400 | 2 | 375/375.0 |
2015 | 2034 | AMINBAZAR 230 | 4034 | AMINBAZAR 400 | 2 | 375/375.0 |
Table 7-24 Capacitors for Period 2010 to 2015
Bus # | Bus Name | KV Level | MVAR |
1126 | KABIRPUR | 132 | 100 |
2045 | OLDRPT | 230 | 180 |
7.7.4 Cost of System Components Added
This section shows the cost of the plan components for the period 2010-2015. The cost of system components used for the cost analysis was provided by PGCB. To perform a cost analysis for the Master Plan the cost of transmission lines, transformers, breakers and manually switched capacitors was taken into account. The cost of transmission lines, transformers, and breakers needed between 2010 and 2015 to keep the transmission system within reliability criteria is shown in Table 7-25.
From 2011-2015, 26 new 132/33 kV substations and connecting 132 kV lines are needed, capable of serving demand of 3,120 MW. The corresponding cost of BDT 15.6 million is incorporated in the costs shown in Table 7-25.
Table 7-25 Cost of Components for 2015
Year | Cost, Million Taka |
2011 | BDT 4,910 |
2012 | BDT 4,910 |
2013 | BDT 4,910 |
2014 | BDT 4,910 |
2015 | BDT 4,910 |
7.8 2020 PLAN
As for years 2010 and 2015, the transmission system for 2020 is the result of back staging the transmission plan developed in the horizon year. The results presented are based on Plan A.
As for years 2010 and 2015 the 2020 load flow base case obtained from the back staging process was tested using load flow and contingency analysis to verify its compliance with reliability criteria. The 2020 substation peak load forecast and the corresponding generation dispatch based on dispatch principles described in Section 7-4 were used for the development of the 2020 load flow base case.
Figure 7-7 shows the 230 KV and 400 KV components of the transmission plan by study year 2020. The load flow data base used for load flow and contingency analysis in 2020 is included in Appendix B.
Figure 7-7 One-Line Diagram of Plan A for Year 2020 (On following page)
117.13
28.26
230.00
78.84
1440 BOGNW
1.013
-9.6
2042 BRPUK23
1.030
-7.8
-32.72
4.28
-32.72
4.28
98.48
15.24
100.00 %
1442 BARPUKR
1.020
-13.1
11.39
86.19
1.26
100.00 %
86.19
1.26
100.00 %
1146 SRIPR132
1.024
-4.2
119.30
39.76
95.83 %
2005 COMI23
0.997
3.1
32.83
-26.93
32.83
-26.93
100.00 %
117.13
28.26
100.00 %
67.19
-34.99
67.19
-34.99
-35.42
-64.04
35.75
59.41
98.15
-48.64
11.39
-48.64
-97.58
2052 SRIPR23
1.024
-2.1
1030 COMI-N
119.30
39.76
95.83 %
119.30
39.76
95.83 %
-208.53
-15.46
-208.53
-15.46
2040 BOG23
1.024
-6.7
117.13
28.26
100.00 %
2036 SRJGNJ
169.37
-155.16
169.37
88.93
-77.19
105.00 %
88.93
-77.19
105.00 %
1139 KASIM
1.000
-4.3
102.16
104.14
-35.42
-64.04
60.26
64.59
93.33 %
60.26
35.75
59.41
108.00 173.08
-56.58
98.15
-56.58
-91.04
34.81
47.39
-97.58
47.39
1.024
0.2
91.89 -184.83
-41.15 27.75
210.79
15.70
210.79
15.70
1.041
-2.5
-11.28
30.93
-11.28
30.93
-168.92
155.86
-168.92
155.86
112.61
-88.32
105.00 %
112.61
-88.32
-155.16
-258.30
232.35
-258.30
232.35
259.73
-225.75
259.73
-225.75
64.59
93.33 %
60.08
-10.78
100 %
1130 GHRASL
1.020
-2.7
-91.04
34.81
7.52
44.22
95.83 %
1201 ASHUGJ
1.018
-0.6
91.89
-41.15
-184.83
27.75
200.49
67.20
400.00
147.97
105.00 %
2039 KASIM23
1.012
80.03
2011 TONG23
60.08
-10.78
7.39
22.39
-86.01
-46.24
200.49
67.20
400.00
147.78
112.61
-88.32
105.00 %
-1.9
77.81
95.00 %
80.03
0.996
-0.6
100 %
97.50 % 2008 ASHU23
1.012
76.30
8.25
-86.01
-46.24
-0.00
-199.15 104.22
77.81
95.00 %
13.96
-13.91
2010 GRSL23
0.988
-87.31
-0.2
7.39
22.39
98.33 %
-0.00
-66.95
37.04
98.75 %
2050 KABIR23
1126 KABIR132
80.03
30.54
-34.23
-0.7
54.95
97.50 %
-0.00
-199.15
-66.95
104.22
1.019
-2.6
1.013
-5.5
77.81
95.00 %
13.96
30.54
-13.91
-34.23
-87.31
54.95
76.30
8.25
98.33 %
-0.00
94.94
29.91
37.04
98.75 %
1125 TONGI
80.03
77.81
95.00 %
82.05 1123 UTTARA132
1104 MANKNG
2001 RAJN23
94.94
29.91
1412 BAGBRI
2030 BAGHA230 1.018
1.016
-2.5
-283.16
32.01
95.83 %
1.021
-1.6
117.69
25.71
97.50 %
1.009
-0.1
76.30
8.25
98.33 %
190.76
-9.84
1.007
15.6
1.020
-4.8
-7.3
2034 AMIN230
54.16
-283.16
301.99
82.05
32.01
117.69
128.90
1003 HATHAZ
190.76
-9.84
-94.50
-38.83
-65.82
15.67
284.84
0.994
1.7
284.31
54.16
2023 MIRPU23
-283.65
-145.55 95.83 %
25.71
97.50 %
77.38
93.75 %
87.73
-61.41
1.019
11.9
76.30
8.25
98.33 %
2002 HATZ23
1.007
15.6
-94.50
-38.83
-65.82
15.67
-47.82
20.30
284.84
-17.86
0.991
0.8
-274.06
82.05
32.01
-176.53
117.69
25.71
128.90
87.73
0.00
-4.66
102.75
33.33
-47.82
284.31
-283.65
24.76
95.83 %
-38.55
97.50 %
77.38
-61.41
97.50 %
2020 ISHRDI
1.006
82.89
-12.53
1401 ISHRDI
20.30
-17.86
274.40
-274.06
-176.53
93.75 %
0.00
-4.66
-6.3
100.00 %
1.012
400.00 284.31
-283.65 -23.56
24.76
-38.55
128.90
150.00
81.23
-81.13
102.75
35.99
41.95
35.99
41.95
82.89
-12.53
100.00 %
82.89
-12.53
100.00 %
-8.3
4034 AMIN400
0.971
6.4
-437.91
217.10
400.00
217.10
291.94
-27.80
98.75 %
20.30
101.48
63.83
95.00 %
101.48
63.83
95.00 %
-17.86
274.40
-23.56
100.72
33.57
95.83 %
100.72
33.57
95.83 %
2022 UTTAR23
0.991
0.4
176.95
41.13
176.95
41.13
2003 MANKNG23
0.993
2.8
394.00
50.04
450.00
139.65
77.38
93.75 %
128.90
77.38
93.75 %
-345.54
-93.34
-345.54
-93.34
34.65
450.00
103.96
450.00
103.96
700.00
66.44
81.23
66.44
-283.06
55.69
-283.06
55.69
-283.06
-68.27
-81.13
-68.27
-72.99
18.27
-72.99
18.27
33.33
97.50 %
102.75
33.33
97.50 %
2044 BMARA23
1.003
-6.4
103.65
73.35
1310 BMARA
41.70
-437.91
41.70
291.94
-27.80
98.75 %
101.48
63.83
95.00 %
100.72
33.57
95.83 %
2004 SIDGJ230
0.999
245.05
14.59
245.05
-244.97
-14.40
-244.97
1107 RAMPRA
1.010
-2.3
2016 RAMP23
0.977
0.8
196.49
150.00
55.69
2025 BARAU23
1.001
15.3
1015 BARAU
1.014
12.8
-35.96
-43.91
-35.96
95.00 %
1.020
-9.4
291.94
-27.80
98.75 %
101.48
63.83
95.00 %
1134 AMINBAZ
1.021
1120 MIRPR132
1.021
-1.6
3.7
14.59
146.34
88.91
-14.40 348.33
109.54
34.65
283.65
-53.54
73.05
85.79
43.51
97.50 %
-43.91
103.65
-0.7
1145 OLDAIR
95.00 %
348.33
362.06
-359.00 283.65
-20.59
73.35
95.00 %
-196.19
98.10
1.020
-1.2
146.34
109.54
23.28
-8.73
-53.54
73.05
-20.59
85.79
157.31 -93.94 -61.19 52.62
88.91
-68.99
138.00
362.06
-359.00 283.65
43.51
-26.88
157.31
-26.88
450.00
22.22
1332 KHULNW
0.06
-93.94
0.06
-178.95
-59.20
-178.95
180.08
-196.19
-61.19
95.00 %
98.10
52.62
95.00 %
146.34
88.91
-60.16
-68.99
-60.16
135.07
225.88
131.67
225.88
23.28
362.06
23.28
-8.73
-359.00
-8.73
-53.54
202.17
-201.73
97.50 %
85.79
1.022 -59.20
58.49
95.00 %
95.00 %
2015 HAR360
131.67
362.06
-359.00 44.94
-44.67
43.51
122.20
20.00
100.00 %
-12.8
94.10
-3.55
180.08
58.49
700.00
338.09
98.10
52.62
146.34
88.91
95.00 %
0.999
3.6
23.28
2026 MD-SKL23
1.017
-8.73
202.17
44.94
90.36
52.85
-201.73
-44.67
97.50 %
1013 KULSH
400.00
155.38
122.20
20.00
100.00 %
119.11
96.13
94.10
-3.55
2013 HASN23
0.996
95.00 %
98.10
52.62
146.34
88.91
95.00 %
-315.58
-201.14
-315.58
316.88
207.58
316.88
19.7
2007 MADNHT23
96.25 %
90.36
52.85
2024 KULSH23
1.001
15.6
1.008
13.5
94.17 %
197.00 122.20
2.4
95.00 %
-201.14
207.58
1.006
96.25 %
20.00
6.52 100.00 %
119.11
96.13
-169.08
169.91
2045 OLD230
1101 HARIPR
1.016
0.1
146.34
88.91
95.00 %
-315.58
-201.14
316.88
207.58
16.4
1005 MADNHT
1.019
-224.82
-155.82 -20.00
20.20
95.00
94.17 %
119.11
-80.29
79.65
0.989
1.1
86.44
36.43
-128.63
-224.82
13.8
21.23 -56.80
37.42
20.20
-3.04
96.13
94.17 %
-169.08
-80.29
169.91
79.65
2006 MAWA23
2029 SHMPR23
-305.14
-80.68
2012 HARI23
0.999
306.41
85.92
86.44
36.43
149.94
-128.63
-155.82 -20.00
37.42
95.00
1.013
1.003
3.6
50.21
87.91
17.79
21.23
-56.80
2049 JHNDHA23
-3.04
119.11
96.13
94.17 %
-249.39
-99.68
3.9
3.1
249.97
101.56
-305.14
-80.68
-305.14
306.41
85.92
306.41
96.25 %
149.94
98.75 %
87.91
17.79
98.75 %
87.91
17.79
98.75 %
1.004
-10.2
94.56
2032 KHUL23
1.029
-9.8
-94.56
-8.36
1113 HASNBD
1.018
-0.4
86.15
-8.72
86.15
-8.72
-249.39
-99.68
86.08
-6.70
100.00 %
1129 SHMPR132
1.000
0.5
249.97
101.56
103.87
9.74
100.00 %
103.87
9.74
-80.68
700.00
81.30
4014 MEGH400
0.970
7.4
194.15
108.34
93.13 %
194.15
108.34
93.13 %
85.92
450.00
217.06
450.00
260.06
50.21
96.25 %
149.94
50.21
96.25 %
149.94
50.21
450.00
71.54
8.36
100.00 %
-94.56
86.15
-8.72
86.08
-6.70
100.00 %
450.00
194.15
108.34
96.25 %
1006 SIKLBHA
2009 SIKBHA23
87.91 -8.36
100.00 %
103.87
68.27
93.13 %
172.00
1.015
0.999
17.79
98.75 %
94.56
8.36
86.15
-8.72
9.74
100.00 %
700.00
194.15
108.34
68.32
14.1
149.94
50.21
96.25 %
18.5
100.00 %
2019 SITLA230
0.996
1114 SITLA132
0.999
103.87
9.74
100.00 %
81.30
93.13 %
439.62
2014 MEGH23
1.015
149.94
1307 JHNDHA132
1.008
-12.8
2051 BAGHER23
1.024
-11.6
2.1
-0.1
-209.45
439.62
-209.45
194.15
108.34
93.13 %
4.6
50.21
96.25 %
7.8.1 Load Flow and Contingency Analysis Results
A number of transmission facilities from the horizon year plan were identified for implementation in the period 2015-2020 in order to maintain the transmission system operating within reliability criteria. Following is a description of those facilities:
- In the Dhaka area we identified the following transmission facilities that need to be considered during the implementation stage for the period 2015-2020. New transmission facilities were identified in the year 2020 to transfer power from generation sites identified by the generation planning process, such as:
− Four 230 KV circuits from the Mawa generation site located southwest of Dhaka to the Hasnabad and Aminbazar substations located in the western side of Dhaka city. Two circuits will connect from Mawa to Hasnabad and the other two from Mawa to Aminbazar
− One 230 kV circuit from Uttara to Tongi and two 230 kV circuits from Mirpur to Uttara. An expansion of the existing Mirpur and Uttara substations will be necessary to accommodate 230 KV facilities.
− One additional 230 kV circuit from Aminbazar to Mirpur.
− Two 230 kV circuits from Hasnabad to Sitalakhya.
− One 230 kV circuit from Meghnaghat to Haripur
- In the Southern region we identified the following transmission facilities that need to be considered during the implementation stage for the period 2015-2020:
− Three 230 KV circuits from the Mandahat/New Sikalbaha generation site to the Mandahat substation. These circuits will support the transfer of power from the proposed Mandahat/New Sikalbaha generation site. This will require an expansion of the Mandahat substation to accommodate new 230 KV facilities.
− The construction of the following 230 KV circuits to keep the transmission system operating within reliability criteria under normal operating conditions with all transmission facilities in service:
- Two 230 KV circuits from Hathazari to Baraulia. This will require an expansion of the Baraulia substation to accommodate new 230 KV facilities.
- Two 230 KV circuits from Baraulia to Kulshi. This will require an expansion of the Kulshi substation to accommodate new 230 KV facilities.
- Two 230 KV circuits from Kulshi to Mandahat.
- Two 230 KV circuits from Mandahat to Hathazari.
Reactive compensation for the period 2015-2020 by the application of shunt capacitors was considered by the Master Plan transmission study. The level of reactive compensation to keep voltages in the 230 KV and 132 KV systems within reliability criteria is 1,590 MVAR in addition to the 1,930 MVAR needed up to year 2015. A table listing the size and location of capacitors for the period 2015-2020 is included in Appendix B.
Table 7-26 shows the level of reactive compensation by region for the 2020 year. The table shows that the level of reactive compensation in general tends to be higher in the major load centers in the country like Dhaka and the Chittagong area in the Southern region.
Table 7-26 Reactive Compensation by Region for 2020
Region | Reactive Compensation (MVAR) |
Southern | 825 |
Dhaka | 1575 |
Central | 180 |
Western | 595 |
Northern | 345 |
Total | 3520 |
The amount of MVAR shown in Table 7-26 above includes the MVAR needed from 2005 up to year 2020. In other words these are cumulative figures.
7.8.2 Summary - Generation Load Losses
Table 7-27 shows active and reactive generation in MW and MVAR, respectively, active and reactive loads in MW and MVAR, respectively, reactive power from capacitors in MVAR, reactive power from transmission lines capacitive effect in MVAR and active and reactive losses by region. The total active losses are about 1.6% of total net load while reactive power losses are substantially higher.
Table 7-27 Generation Load and Losses by Region 2020
| GENERATION | LOAD | CAPAC- ITORS | LINE CHARGING | LOSSES |
REGION | MW | MVAR | MW | MVAR | (MVAR) | (MVAR) | MW | MVAR |
SOUTHERN | 2850 | 749 | 2771.3 | 1341.9 | 845 | 195.3 | 58.4 | 645.2 |
DHAKA | 6678 | 2423.3 | 6375.9 | 3087.2 | 1518.7 | 677.9 | 68.7 | 1335.9 |
CENTRAL | 1088 | 347.9 | 1164 | 563.6 | 182.6 | 64.9 | 21.3 | 167 |
WESTERN | 1447 | 288.2 | 1624.7 | 786.9 | 624.7 | 189.9 | 35 | 261.8 |
NORTHERN | 1730 | 626.1 | 1636.4 | 792.0 | 361.6 | 260.3 | 37.2 | 374 |
TOTALS | 13793 | 4434.5 | 13572.4 | 6571.6 | 3532.6 | 1388.3 | 220.6 | 2783.9 |
7.8.3 System Components Added
The 230 KV transmission lines of the transmission plan for the period 2015-2020 are shown in Table 7-28. Table 7-29 shows transformers for 230/132 KV substations. Table 7-30 shows capacitors larger than 100 MVAR. Tables listing all facilities are included in Appendix B.
Table 7-28 Transmission Lines for Period 2015 to 2020
Year | Bus # | Name | Bus # | Name | # Added CKT | KM |
2020 | 1006 | HALISHAB 132 | 1017 | JULDA 132 | 1 | 5.00 |
2020 | 1020 | FENI 132 | 1021 | CHOWMUHUNI 132 | 1 | 32.00 |
2020 | 1101 | HARIPUR 132 | 1102 | SIDDHIRGANJ 132 | 1 | 1.50 |
2020 | 1101 | HARIPUR 132 | 1109 | MATUAIL 132 | 1 | 10.00 |
2020 | 1102 | SIDDHIRGANJ 132 | 1105 | ULLON 132 | 1 | 16.00 |
2020 | 1104 | MANIKNAGAR 132 | 1108 | NARINDA 132 | 1 | 3.00 |
2020 | 1105 | ULLON 132 | 1106 | DHANMONDI 132 | 1 | 5.50 |
2020 | 1105 | ULLON 132 | 1107 | RAMPURA 132 | 1 | 4.00 |
2020 | 1107 | RAMPURA 132 | 1116 | GULSHAN 132 | 1 | 7.00 |
2020 | 1107 | RAMPURA 132 | 1140 | MADERTK 132 | 1 | 2.00 |
2020 | 1111 | SHAMPUR 132 | 1129 | SHAMPUR TAP 132 | 1 | 2.50 |
2020 | 1113 | HASNABAD 132 | 1118 | KAMRNGICHAR 132 | 1 | 11.00 |
2020 | 1120 | MIRPUR 132 | 1123 | UTTARA 132 | 1 | 13.00 |
2020 | 1122 | KALYANPU 132 | 1134 | AMINBAZA 132 | 1 | 3.00 |
2020 | 1123 | UTTARA 132 | 1125 | TONGI 132 | 1 | 9.00 |
2020 | 1124 | BASUNDHARA 132 | 1125 | TONGI 132 | 1 | 8.00 |
2020 | 1126 | KABIRPUR 132 | 1128 | TANGAIL 132 | 1 | 50.00 |
2020 | 1126 | KABIRPUR 132 | 1127 | MANIKGAN 132 | 1 | 26.00 |
2020 | 1126 | KABIRPUR 132 | 1139 | KASIMPUR 132 | 1 | 11.00 |
2020 | 1130 | GHORASAL 132 | 1133 | BHULTA 132 | 1 | 20.00 |
2020 | 1131 | NARSHIND 132 | 1136 | PURBCHL 132 | 1 | 13.00 |
2020 | 1134 | AMINBAZA 132 | 1141 | MOHMPUR 132 | 1 | 12.00 |
2020 | 1142 | UNIVERSITY 132 | 1145 | OLDARPT 132 | 1 | 6.50 |
2020 | 1143 | CANTOMNT 132 | 1145 | OLDARPT 132 | 1 | 6.00 |
2020 | 1201 | ASHUGANJ 132 | 1210 | B-BARIA 132 | 1 | 12.00 |
2020 | 1210 | B-BARIA 132 | 1211 | SHAJIBAZAR 132 SRIMONGAL 132 | 1 | 40.00 |
2020 | 1211 | SHAJIBAZAR 132 SRIMONGAL 132 | 1212 | 1 | 36.40 |
2020 | 1212 | 1213 | FENCHUGA 132 | 1 | 49.20 |
2020 | 1323 | BHANDARIA 132 | 1324 | BAGERHAT 132 | 1 | 32.00 |
2020 | 1403 | NATORE 132 | 1405 | RAJSHAHI 132 | 1 | 40.00 |
2020 | 1415 | BOGRA 132 | 1440 | BOGRANEW 132 | 1 | 2.00 |
2020 | 1213 | FENCHUGA 132 | 1214 | FENCHUGA PS 132 | 2 | 3.50 |
2020 | 2002 | HATHAZARI 230 | 2007 | MANDAHAT 230 | 1 | 9.00 |
2020 | 2011 | TONGI 230 | 2022 | UTTARA 230 | 1 | 9.00 |
2020 | 2012 | HARIPUR 230 | 2014 | MEGHNAGH 230 | 1 | 11.60 |
2020 | 2023 | MIRPUR 230 | 2034 | AMINBAZA 230 | 1 | 10.00 |
2020 | 2002 | HATHAZARI 230 | 2025 | BARAULIA 230 | 2 | 12.00 |
2020 | 2006 | MAWA 230 | 2013 | HASNABAD 230 | 2 | 30.00 |
2020 | 2006 | MAWA 230 | 2034 | AMINBAZA 230 | 2 | 40.00 |
2020 | 2007 | MANDAHAT 230 | 2024 | KULSHI 230 | 2 | 12.70 |
2020 | 2013 | HASNABAD 230 | 2019 | SITALAKHYA 230 | 2 | 12.00 |
2020 | 2022 | UTTARA 230 | 2023 | MIRPUR 230 | 2 | 13.00 |
2020 | 2024 | KULSHI 230 | 2025 | BARAULIA 230 | 2 | 12.90 |
2020 | 2007 | MANDAHAT 230 | 2026 | MAND/SIKALB 230 | 3 | 30.00 |
Table 7-29 Transformers for Period 2015 to 2020
Year | Bus # | Name | Bus # | Name | # Added Trnsfrmrs | MVA |
2020 | 1006 | SIKALBAH 132 | 2009 | SIKAL23 230 | 1 | 225/225.0 |
2020 | 1013 | KULSHI 132 | 2024 | KULSHI 230 | 3 | 225/225.0 |
2020 | 1015 | BARAULIA 132 | 2025 | BARAULIA 230 | 3 | 225/225.0 |
2020 | 1101 | HARIPUR 132 | 2012 | HARIPUR 230 | 1 | 225/225.0 |
2020 | 1123 | UTTARA 132 | 2022 | UTTARA 230 | 3 | 225/225.0 |
2020 | 1126 | KABIRPUR 132 | 2050 | KABRPR23 230 | 1 | 225/225.0 |
2020 | 1134 | AMINBAZAR 132 | 2034 | AMINBAZAR 230 | 1 | 225/225.0 |
2020 | 1307 | JHENIDA 132 | 2049 | JHENIDA 230 | 2 | 225/225.0 |
2020 | 1440 | BOGRANEW 132 | 2040 | BOGRA 230 | 1 | 225/225.0 |
2020 | 2014 | MEGHNAGHAT 230 | 4014 | MEGHNAGHAT 400 | 2 | 375/375.0 |
2020 | 2034 | AMINBAZAR 230 | 4034 | AMINBAZAR 400 | 2 | 375/375.0 |
Table 7-30 List of Capacitors Larger Than 100 MVAR for Year 2020
Bus # | Bus Name | KV Level | MVAR |
1126 | KABIRPUR | 132 | 360 |
1127 | MANIKG | 132 | 135 |
1128 | TANGAL | 132 | 135 |
1306 | JESORE | 132 | 180 |
2002 | HATHAZARI | 230 | 300 |
2011 | TONGI | 230 | 270 |
7.8.4 Cost of System Components Added
This section shows the cost of the plan components for the period 2015-2020 for Plan A. The figures shown in the table below include the cost of transmission lines, transformers, breakers, and manually switched capacitors.
The cost of transmission lines, transformers, and breakers needed between 2015 and 2020 to keep the transmission system within reliability criteria is shown in Table 7-31.
From 2016-2020, 30 new 132/33 kV substations and connecting 132 kV lines are needed, capable of serving demand of 3,600 MW. The corresponding cost of BDT 15.6 million is incorporated in the costs shown in Table 7-31.
Table 7-31 Cost of Components for 2020
Year | Cost, Million Taka |
2016 | BDT 6,412 |
2017 | BDT 6,412 |
2018 | BDT 6,412 |
2019 | BDT 6,412 |
2020 | BDT 6,412 |
Sensitivity analysis was conducted for year 2020 by modifying the dispatch shown in Table 7-2. The sensitivity analysis was conducted to test the capability of the transmission plan to evacuate the system generation without violation of criteria. For 2020 two sensitivities were conducted as follows:
- In the Dhaka area existing generation at Ashuganj and Ghorasal, which was off- line in the basic dispatch used to develop the transmission plan in 2025, was dispatched at full capability in place of generation in the proposed Mawa generation complex located southwest of Dhaka and in Siddhirganj generation center. Load flow analysis and contingency analysis were conducted indicating that the plan was within criteria. Results are shown in Appendix B.
- In the Northern Region existing generation at Babhabari, which was off-line in the basic dispatch used to develop the transmission plan in 2025, was dispatched at full capability in place of generation in the proposed Mawa generation complex located southwest of Dhaka. Load flow analysis and contingency analysis were conducted indicating that the plan was within criteria. Results are shown in Appendix B.
Section 8 Economic and Financial Analysis
The TOR’s Component B, Task 3 (vi) requires the consultant to “Assist BPDB, PGCB, and PMU to prepare financial projections up to the year 2025, with emphasis on cash flows for the recommended power system expansion plan, and conduct economic analyses to determine if the proposed development program is justified.”
We note that this differs from the more detailed financial analyses, plans, and projections required by Component C, items 4 (iv), (v), and (vi). Those items will be addressed in Phase 2 of this study.
Our economic analysis demonstrates how the general approach to generation and transmission planning produces proposed projects and a development program that is justified on an economic basis. The financial analysis shows the annual expenditures to 2025 required by the master plan program of generation and transmission additions.
8.1 ECONOMIC ANALYSIS
8.1.1 Generation Expansion Plan
The objective of the generation expansion planning process is to develop a least cost plan subject to certain constraints. The least cost plan includes a schedule of additions of different generating plants of specific size, technology, fuel, efficiency, and other parameters. By least cost we mean that replacing any of these additions with a different plant, or changing the schedule of additions, will increase the overall cost of the plan.
Accordingly, the plan itself is economically justified because no other plan costs less. Each unit in the plan is economically justified because replacing it with a different unit, or not replacing it at all, increases overall costs.
We know that the plan and the individual units are least cost because the program doing the calculations has evaluated tens of thousands of the most likely possibilities and selected the lowest cost of all those. It has tried adding or removing individual units or changing the time they are added to the plan, and in each case found that doing so increases costs compared to the least cost plan.
A crucial question is how much generation is needed to achieve a least cost plan. Every additional plant increases the reliability of the system and permits more energy to be delivered to customers. At some point the cost of adding another plant is more than the benefit of delivering the corresponding additional energy to customers. Section 6 discusses this subject in detail and we will not repeat that discussion here. In summary, the planning process undertaken in this study quantifies the value of reliability. It balances the benefit of increased reliability against the cost of achieving it, and adds new generation until a balance is achieved where adding one more plant would increase costs more than benefits, and removing one plant would reduce costs less than benefits.
To illustrate that the planning process, we will first summarize the discussion in Section 6 on screening analysis. Screening analysis assures that the most economical power plants at each capacity factor are among the candidates that could be included in the least cost plan. By eliminating clearly uneconomic candidates it also makes the optimization process more
efficient. Next we will describe how production simulation and system optimization using the WASP-IV program establishes the least cost plan. Finally, we will address some of the constraints and other issues with the potential to affect the results.
8.1.1.1 Screening Analysis
In screening analysis the total annual energy production cost for a generating unit, including all capital-related and operating expenses, expressed in $/KW-year, is plotted as a function of the capacity factor. The vertical axis intercept (zero capacity factor) represents the fixed costs that do not vary with the energy output of the unit. A typically straight line shows the increase in annual costs with increasing capacity factor with a slope proportional to the unit’s variable costs.
The least cost curve is the set of line segments that are the lowest cost alternative at each capacity factor. Figure 8-1 shows the least-cost curve for this study. It shows that the lowest cost alternative is ENS (energy not served, in other words demand not met due to load shedding) from zero to 1.5% capacity factor, a 150 MW simple cycle gas turbine from 1.5% to about 16%, and a 700 MW CC for 16% and higher. The 450 MW CC is not on the least cost curve. Because the 700 MW CC has not yet been implemented in Bangladesh, we have assumed in the Base Case that such a unit could not come on line before 2018. Until then the 450 MW unit would be the least cost option for about 26% capacity factor and higher. The SCGT would be least cost from about 1.5% to 26%. We will discuss other technologies below.
Figure 8-1 Least Cost Curve – Base Case
Other factors such as forced outage rates, unit sizes, and system reliability are not treated directly with screening curves. Nevertheless, one would expect that WASP would select the 700 MW CC for plants that operate above about 16% lifetime capacity factor, the 150 MW SCGT for plants that operate from about 1.5% to 16% capacity factor, and add no plant if it would operate below 1.5% capacity factor.
Figure 6-2 demonstrated that all other technologies suitable for base load applications have cost curves well above those of the CC units at all capacity factors. All the other technologies suffer from higher capital costs and lower efficiencies. The coal plant offsets the lower efficiency with fuel costs lower by about the same amount. The nuclear plant’s fuel costs are much lower, but that does not nearly compensate for the higher capital costs.
Figure 8-2 compares technologies best suited for peaking duty, combining the results shown in Figures 6-3 and 6-4. The two SCGT options, 100 MW and 150 MW units, are close in both capital cost and efficiency, so their cost are close throughout the range shown. We include three cost of unserved energy lines, corresponding to KWH values of $1.00, $0.43, and $0.20. The highest cost places more value on reliability, resulting in a lower crossing point vs. the cost of new SCGT units – at about 0.5% equivalent capacity factor for the SCGT units. The lowest cost results in a crossing point of about 3.5%.
Figure 8-2 Comparison of Peak Load Technologies
In summary, the gas-fired SCGT and CC technologies are clearly the lowest cost options for service at capacity factors from under 5% and above. Very low capacity factors for unserved energy correspond to very rare events, as discussion further below will indicate. For these rare events, the economic choice is to fail to meet demand and accept a small amount of load shedding.
8.1.1.2 Production Simulation and System Optimization Analysis
The WASP-IV program takes into account factors that screening analysis cannot. It considers such factors as:
- Planned maintenance, and it establishes an optimal schedule for each unit’s annual maintenance considering system needs and the maintenance needs of other units.
- Forced outages.
- The expected amount of energy not served and its cost.
- Loss-of-load-probability (LOLP) criteria.
- Unit size. The lowest cost option for (say) base load duty may be larger than one year’s load growth, and thus provide some unneeded capacity for a period.
- Spinning reserve requirements.
The program is designed to determine the least cost generation expansion plan that fulfills a specified LOLP criterion. It does so by evaluating all the costs of system operation, including fuel, O&M, the capital costs of new plants, and the cost of unserved energy. It calculates the present worth of all these costs at a reference point such as the study period start date.
WASP-IV uses an iterative method to add and delete resources, by calculating and comparing the lifecycle costs and benefits of each resource option for every year for which the resource is available. Adding a new plant to the resource mix adds its fuel, O&M, and capital costs to the system. It reduces fuel and O&M costs at other plants, and reduces the costs associated with energy-not-served. When these cost reductions are large enough to offset the operating and investment-related costs of the new plant, the new plant may be added to the resource plan.
WASP evaluates tens of thousands of alternative resource plans using a dynamic programming approach to reach an optimal solution quickly. The resulting resource plan is the least cost of all.
The new generating plants (beyond the committed resources) added in the Base Case resource plan include only natural gas-fueled 150 MW SCGT units, 450 MW CC plants, and 700 MW CC plants. This entirely consistent with what one would expect from the screening analysis. It is also not surprising because a similar pattern occurs wherever relatively low cost natural gas is available.
The approach is logical and comprehensive, but it is difficult to prove in a mathematical sense that the resulting resource plan is lowest in cost of all possible plans. We can make the following observations:
- The resulting resource plan is stable in the program has determined that adding or subtracting any resource will increase overall costs.
- The new plants operate within their optimal capacity factor range as indicated in the screening analysis.
Screening analysis is based on lifetime average capacity factors. In a given year a unit’s capacity factor could fall outside the preferred range. In the first years of the study period the
system is unbalanced due to inadequate generation and other factors. By 2020 and 2025 the system is balanced and has a stable mix of generation. Table 8-1 shows the capacity factors of different unit classes in 2020 and 2025.
Table 8-1 Unit Class Capacity Factors
| 2020 | 2025 |
150 MW SCGT | 5.2% | 5.8% |
450 MW CCGT | 53.2% | 53.2% |
700 MW CCGT | 79.0% | 73.0% |
Table 8-1 places the unit class capacity factors where one would expect them to fall. The more cost-effective 700 MW CC has the highest capacity factor, the 450 MW CC units installed before 2018 have lower capacity factors still well within their economic range, and the 150 MW SCGT unit class has a low capacity within its economic range.
Figure 8-3 shows ENS for the years 2005 – 2025 expressed in GWH/year and as the equivalent capacity factor for a 150 MW SCGT. In other words, if all the ENS could be replaced by energy from a single 150 MW SCGT unit, that unit would have the capacity factors shown.
500 | | 25% |
450 | | |
400 | Energy | 20% |
350 | Capacity Factor | |
Figure 8-3 Energy Not Served and Equivalent Capacity Factor
Figure 8-3 shows that if another 150 MW SCGT were to be added to the expansion plan after 2008, it would operate below 2% capacity factor even if it could replace all the ENS each year. Thus the ENS is within its economic range. Between 2005 and 2008 ENS and corresponding equivalent capacity factors are much higher. During that period the system is moving toward having an adequate level of generation, which it achieves in 2009.
The ENS values shown, after 2008 all below about 20 GWH/year and from 0.01 to 0.03% of energy demand, are far below estimates of load shedding since 1994. Depending on the year, these estimates range from 104 GWH/year in 2003 to 826 GWH/year in 1997, or 0.6% to 7%
of annual energy demand. Naturally, at peak demand time the historical impact of lead shedding is much more severe, ranging from 10% to 20% of peak demand.
In other words all three cost of energy not served values produce much more reliable systems than has been the case historically.
Using our basic assumptions, we conclude that the plan as a whole is a least cost plan, and its individual new projects are also least cost. Therefore we conclude that the proposed generation development program is justified.
8.1.1.3 Constraints and Other Issues
The projects and plan are least cost within the framework of the constraints and other input data assumptions. The plan is based on forecasts of future conditions that are bound to be less than perfectly accurate. This cannot be avoided, but can be analyzed by developing scenarios to determine how the generation expansion plan would change in response to changes in inputs.
Depending on the results, the policy response might be to take risk-mitigation measures that at some cost would protect against unfavorable outcomes. The focus clearly should be on the how near term decisions would be affected.
Typically the resource plan results confirm what one would expect, at least in terms of the general direction of any changes. For example, using High Case rather than Base Case load growth results in many more units being added to the resource plan. The Low Case has fewer units. The question is what to do about that result.
Below we discuss the implications of the results for three of the most important inputs.
Load Growth
Table 8-2 shows the annual and cumulative additions of the new units for the three load growth scenarios. There is a significant difference among the scenarios as early as 2008. For the Base Case, two 150 MW SCGTs come on line in 2008. For the High Case, two 450 MW CCs come on line in 2008. For the Low Case, no new units are built by 2008. In other words the choices today are to proceed very quickly with the 150 MW SCGTs, proceed immediately with the 450 MW CCs, or continue only with preliminary steps for the time being.
We believe the Base Case is more likely than the other two cases. It more closely represents a continuation of the trend of the last 10 years. Therefore we believe that any course of action should include starting the process resulting in implementation of two new 150 MW SCGTs by 2008. However, in all three cases by 2009 the plans call for at least one 450 MW CC. By 2010 the plans call for at least one 150 MW SCGT and two 450 MW CCs. Given the possibility of delays in approval and construction of new units, it would be prudent to undertake all measures short of final commitment for at least one 450 MW CC by 2009 and two such units by 2010. Final decisions on these units will have to be made in the relatively near future to meet the plan’s on-line dates.
Table 8-2 Resource Plan Additions for Load Growth Scenarios
| Annual Additions, Number of Units, for Demand Growth Scenarios |
| Low | Base | High |
Year | 150 MW CT | 450 MW CC | 150 MW CT | 450 MW CC | 150 MW CT | 450 MW CC |
2005 | 0 | 0 | 0 | 0 | 0 | 0 |
2006 | 0 | 0 | 0 | 0 | 0 | 0 |
2007 | 0 | 0 | 0 | 0 | 0 | 0 |
2008 | 0 | 0 | 2 | 0 | 0 | 2 |
2009 | 0 | 1 | 0 | 1 | 0 | 1 |
2010 | 1 | 1 | 0 | 2 | 2 | 2 |
2011 | 1 | 1 | 1 | 1 | 0 | 2 |
2012 | 0 | 1 | 0 | 2 | 0 | 3 |
| | | | | | |
| Cumulative Additions, Number of Units, for Demand Growth Scenarios |
| Low | Base | High |
Year | 150 MW CT | 450 MW CC | 150 MW CT | 450 MW CC | 150 MW CT | 450 MW CC |
2005 | 0 | 0 | 0 | 0 | 0 | 0 |
2006 | 0 | 0 | 0 | 0 | 0 | 0 |
2007 | 0 | 0 | 0 | 0 | 0 | 0 |
2008 | 0 | 0 | 2 | 0 | 0 | 2 |
2009 | 0 | 1 | 2 | 1 | 0 | 3 |
2010 | 1 | 2 | 2 | 3 | 2 | 5 |
2011 | 2 | 3 | 3 | 4 | 2 | 7 |
2012 | 2 | 4 | 3 | 6 | 2 | 10 |
Gas Price
We developed the Limited Gas scenario to address the possibility that new gas will not be developed to the extent we assume for the Base Case. The price of the gas does not change. WASP-IV inputs for this scenario permit no new base-load gas-fueled units after 2015. New peaking SCGTs are permitted. New coal plants replace what were, in the Base Case, 450 MW and 700 MW combined cycle plants. This result of course is a crucial impact on the long-term resource plan. By itself, however, it has no near-term impact because decisions on what fuels are available for plants beginning operation after 2015 should not be made now, but rather after about 2010 based on the better information available then.
Gas price could influence both near-term and long-term decisions. For the Base Case, we used a levelized gas price of $3.02 per GJ based on 75% of the forecast levelized HFO price of $4.03/GJ. Section 4 explains our rationale for using this price. In comparison, today’s price Taka 73.91/1,000 cubic feet is equivalent to about $1.25/GJ. We evaluated gas price with screening analysis, using levelized gas prices per GJ of $1.25 (Low), $3.02, and $4.03 (High). Figure 8-4 compares annual costs based on the High gas price. The High gas price makes the coal plant more competitive compared to the gas options, and the CC more competitive compared to the SCGT. The coal plant’s annual cost matches that of the 70 MW CC at 90% capacity factor, and is below that of the 450 MW combined cycle at capacity factors above about 60%. It is never on the least cost curve with Base Case gas prices.
The lowest cost alternative is ENS from zero to 1.5%, a 150 MW simple cycle gas turbine from 1.5% to about 13%, the 700 MW CC from 12% to 90%, and the coal plant above 90%. As a practical matter a capacity factor above 90% would be extremely unusual for a coal plant, so the larger CC would be the choice for all realizable capacity factors above 13%.
Until the 700 MW CC is assumed to be available in 2018, the 450 MW CC is the lowest cost alternative from about 20% to 61%, and the coal plant above 61%.
Figure 8-4 Least Cost Curve – High Gas Prices
Figure 8-5 compares annual costs based on the Low gas price. The Low gas price makes the gas options more competitive compared to the coal plant, and SCGT option more competitive compared to the CC. The coal plant is never on the least cost curve. The SCGT is least cost from 1.4% to 38% capacity factor compared to the 700 MW CC and from 1.4% to 70% compared to the 450 MW CC.
Table 8-3 summarizes these results. In Section 4 we explained our rationale for selecting the Base Case gas price. Considering that the current price is well below the price we have used in analysis, the High gas price scenario seems unlikely. Furthermore, the higher price does not change the results as significantly as the lower price. The SCGT is the least cost option up to 12% capacity factor instead of 16% in the Base Case. The 700 MW CC is least cost for realizable capacity factors above 12%. If the 700 MW CC is not available, some coal plants would enter the resource plan for high capacity factor operation.
| Capacity Factors for Which Generation Option is Least Cost When 700 MW CC is Available | | Low Gas Price | Base Gas Price | High Gas Price | Energy Not Served | 0% to 1.4% | 0% to 1.5% | 0% to 1.5% | 150 MW SCGT | 1.4% to 38% | 1.5% to 16% | 1.5% to 12% | 450 MW CC | None | None | None | 700 MW CC | 38% and higher | 16 % and higher | 12% to 90% | 500 MW Coal Plant | None | None | Above 90% | | | | | | Capacity Factors for Which Generation Option is Least Cost When 700 MW CC is Not Available | | Low Gas Price | Base Gas Price | High Gas Price | Energy Not Served | 0% to 1.4% | 0% to 1.5% | 0% to 1.5% | 150 MW SCGT | 1.4% to 70% | 1.5% to 26% | 1.5% to 20% | 450 MW CC | 70% and higher | 26% and higher | 20% to 61% | 700 MW CC | Not Available | Not Available | Not Available | 500 MW Coal Plant | None | None | 61% and higher |
| |
Figure 8-5 Least Cost Curve – Low Gas Prices Table 8-3 Least Cost Options at Different Gas Prices
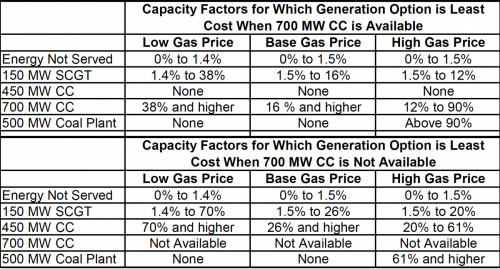
With the Low gas price, far more SCGTs would be built. Until the 700 MW CC became available, most new units would be 150 MW SCGTs. Gas price to some extent is a policy choice of the GOB. In our opinion, using a low gas price in analysis and building primarily SCGTs as a result would be wasteful of a resource that has high long-term value. However, this is a subjective viewpoint not necessarily shared by policy makers.
Reliability
We have noted that a fundamental trade-off in the generation expansion planning process is between cost and reliability. Our analysis uses two reliability measures. First, a minimum reliability of one day per year or less loss-of-load-probability is required. Second, WASP-IV performs an economic test that adds units until the cost of the next additional unit would be more than its benefits. That unit is not added. The benefits include its impact on reducing ENS as well as on reducing fuel costs.
There are two main questions. First, does the LOLP constraint require more units than the economic test? If it does, then the generation expansion plan may not be least cost. Second, what is the best value for unit cost of ENS?
To address these questions we evaluated six scenarios. In one set of three we applied the LOLP criterion. In the other set of three, we did not. Of the three scenarios in each set, one uses a Base Case unit cost of ENS of $0.43 per KWH. Another uses a High COENS of $1.00 per KWH. The third uses a Low COENS of $0.20 per KWH Table 8-4 summarizes the scenarios.
Table 8-4 Summary of COENS Scenarios
| NAMES OF SCENARIOS |
COENS | LOLP Criterion Applied | LOLP Criterion Not Applied |
Base = $0.43/KWH | LOLP Criterion, Base COENS | No LOLP Criterion, Base COENS |
High = $1.00/KWH | LOLP Criterion, High COENS | No LOLP Criterion, High COENS |
Low = $0.20/KWH | LOLP Criterion, Low COENS | No LOLP Criterion, Low COENS |
Figure 8-6 plots the MW of installed capacity for all six scenarios. Figure 8-6 shows the following:
- The installed capacity is the same for all scenarios for the first few years. This occurs because only plants already in progress can be built before the end of that period, and we do not begin to apply the LOLP criterion in any case until 2009.
- There is almost no difference among the scenarios where the LOLP criterion is applied (solid lines). This means that the LOLP criterion is governing in determining the level of reliability.
- All the scenarios where the LOLP criterion is applied show more installed capacity than all the scenarios where it is not. This too demonstrates that the LOLP criterion is governing in determining the level of reliability.
- For the scenarios where the LOLP criterion is not applied (dotted lines), the results are as expected. In other words, where economics alone governs, higher COENS results in more installed MW.
- In 2025 the difference between the Base Case with LOLP criterion applied and the Base Case where it is not applied reaches its maximum or 1,270 MW in 2024 and averages 804 MW over 2009 – 2025. Roughly speaking, for the Base Cases by 2025 the annual fixed cost of about 800 MW of capacity is the price paid for maintaining higher reliability by applying a LOLP criterion instead of only an economic criterion.
- However, for the High Cases the difference is only 350 MW by 2025. At slightly higher values of COENS there would presumably be no difference, and the economic criterion would be governing.
- Where the LOLP criterion is not applied, the range in installed capacity is 1,500 MW from the Low COENS case (20,190 MW) to the High COENS case (21,690).
Figure 8-6 LOLP Expectation for Cost of ENS Cases
It is common for criteria such as LOLP, reserve margin, or largest single contingency to be applied even when economic criteria are applied. Part of the reason for this is historical.
Planners applied deterministic criteria such as reserve margin or largest single contingency before the probabilistic techniques were developed. LOLP is a probabilistic technique that came into common use before the probabilistic/economic COENS approach. Maintaining tools that had proved useful for decades is natural.
However, another reason is that the unit cost of energy not served is uncertain. It is not known with precision for any customer, is no doubt different for different groups of customers, and as used in our system optimizations is the same for all customers. The analysis above indicates that the Base Case resource plan is consistent with a COENS value slightly above $1.00/KWH.
If a value slightly above $1.00 per KWH is appropriate for COENS, then the resource plan is least cost. The discussion of Section 6 indicates that values in the range $0.20 to $1.00 per KWH are reasonable. If a lower value is more appropriate, then the resource plan provides more capacity than a strict economic approach would dictate.
This suggests two areas worthy of more investigation. The first is to evaluate whether the LOLP criterion should be applied. The second is to develop a Bangladesh-specific estimate of the most appropriate value for COENS. In the near term, however, there is no doubt that the system needs more capacity and aggressive action should be taken to achieve that result.
Summary
From the discussion above, we draw the following conclusions:
- We believe that any course of action should include starting the process resulting in implementation of two new 150 MW SCGTs by 2008, as required using the Base Case demand forecast. It would be prudent to undertake all measures short of final commitment for at least one 450 MW CC by 2009 and two such units by 2010. Final decisions on these units will have to be made in the relatively near future to meet the plan’s on-line dates.
- This analysis used a liquid fuel based price for gas in establishing the generation expansion plan. Using substantially lower prices such as today’s price would lead to many more SCGTs and fewer CCs.
- The Base Case generation expansion plan provides a least-cost level of reliability if the appropriate value for COENS is slightly above $1.00 per KWH. If a more appropriate value is lower, for example our Base Case value of $0.43 per KWH, then the resource plan provides more capacity than a strict economic approach would dictate.
- Further investigation is merited to evaluate whether the LOLP criterion should be applied, and to develop a Bangladesh-specific estimate of the most appropriate value for COENS.
8.1.2 Transmission Expansion Plan
The objective of the generation expansion planning process is to achieve a least cost solution. The costs and benefits of reliability are taken into account explicitly. The objective of the transmission planning process is to develop a system that meets technical planning criteria.
There is no explicit tradeoff of cost and reliability, but the criteria were developed over time taking that tradeoff into account. Within that framework, however, the process is consistent with least cost planning.
Section 7 describes the criteria used for transmission planning in this study. They are designed to ensure the sound operation of the system during normal and contingency conditions with respect to voltages and the loading of equipment.
The starting point for developing the transmission expansion plan is the existing system. PGCB provided a system configuration for the year 2010 that was used as a starting point for planning. Using this 2010 system as a base, we developed forecasts of loads at all the 2010 substations and generation at all existing and new sites for the years 2015, 2020, and 2025.
The first major step was to develop the 2025 transmission system. Loads and generation in 2025 are much higher than in 2010, so without massive expansion of the 2010 system the planning criteria would be violated almost everywhere. Using their experience and judgment, the transmission planning specialists added components in an effort to bring the system into compliance with the planning criteria, including:
- Connecting generation at new or expanded existing sites to the main transmission system.
- Adding new transformers and circuits.
- Placing reactive support where it would be most effective.
In the course of doing this, the specialists faced numerous decisions on resolving problems (criteria violations) in different areas. They were aware of and took into account the cost implications in making their choices, but did not perform economic comparisons directly. Eventually they established a tentative Base Case for 2025 and subjected it to additional analysis, to confirm its performance with respect to contingencies, stability, and short circuit. Where problems appeared their solution usually involved additional components. Eventually this process produced a final 2025 Base Case.
The point is that the 2025 Base Case represents a least cost solution in that it incorporates the minimum amount of system expansion that meets the technical planning criteria. The planning criteria take the tradeoff of the cost and benefits of reliability into account, and they are similar or identical to those used around the world in well-designed and operated systems.
The transmission planning specialists then developed the systems for 2020, 2015, and 2010 in sequence by working backward from the 2025 Base Case. Using the loads and generation for 2020 (for example), they removed transmission system components from the 2025 Base Case until the planning criteria were just met. This produced the 2020 Base Case, which was then subject to a similar process to develop the 2015 Base Case, and then the 2010 Base Case.
Because the 2010 Base Case was established in this manner, it is not identical to the 2010 system developed by PGCB, although it is similar.
Accordingly, for each of the years studied the transmission expansion plan incorporates the minimum amount of system expansion that meets the technical planning criteria and thus is least cost from that viewpoint.
The advantage of this “back staging” approach compared to developing the transmission system in chronological order (first for 2010, then for 2015, etc.) is that one avoids installing components that later become redundant, or worse, committing to a course that is well below optimal for the long term. This contributes to system development that is least cost from a long-term perspective.
We did conduct an economic analysis to determine the most economic option between Plan A, which uses 230 kV as the highest voltage level (apart from the 400 kV committed project) and Plan B, which adds additional 400 kV facilities. As indicated in Section 7, Plan A has a lower cost than Plan B by about 2%. Although higher voltage transmission facilities can handle larger power flows and operate with lower loss levels, Bangladesh is not a large geographic area, is not interconnected with neighboring countries, has and will have substantial generation located close to the larger load centers like Dhaka and Chittagong, and thus will not be moving large blocks of power large distances around the country. Based on the aforementioned statements, we selected Plan A as the appropriate approach for transmission development over 2005 – 2025: continuing to use 230 kV as the highest voltage level for new transmission facilities needed to continue the expansion of the transmission system in an economical and reliable fashion.
Another important issue is whether to locate new generation at load centers, to minimize electric transmission costs, or to site new generation near gas fields, to minimize gas transmission costs, or (more likely) some combination. Determining a joint least cost gas and electric transmission development scheme is beyond the scope of this study, but we believe the topic should be addressed as a priority matter.
Based on the discussion above, but setting aside the question of a joint least cost gas and electric transmission development scheme, we conclude that the proposed transmission development program is justified.
8.2 FINANCIAL ANALYSIS
The projected cash flows for the generation and transmission expansion plans to 2025 under the “Base Case, Sufficient Gas” Scenario are presented in sub-section 8.2.1 below. The data are based on the Master Plan results presented in the Interim Report. There would be little difference if we used data based on the revised Master Plan results presented in this report. Detailed financial results based on the revised Master Plan are presented in a companion volume of this report: Draft Final Report, Component C: Preparation of Investment Projects, Volume 2: Financial Issues. The projections below are presented in constant 2005 US dollars and are prepared on the following basis:
- Investments (Public and Private)
− Ongoing and committed investments, including rehabilitation, based on contract prices or latest BPDB/PGCB cost estimates. Financing costs are excluded.
− Planned investments as per the PSMP. Financing costs are excluded. The investment program covers all generation plants and extension and reinforcement of the transmission network required to be commissioned by 2025 under the projected “Base Case” load growth.
- Fuel costs based on the two alternatives shown in Table 8-5.
- Operations and maintenance (O&M) costs based on standard cost estimates.
The costs shown do not include any capital-related costs associated with the existing system, or any costs associated with the distribution system, which are not part of the Master Plan.
Cash inflows necessary to support these outflows will obviously be necessary. Identifying the source and amount of these inflows is beyond the scope of this project.
Table 8-5 Fuel Price Scenarios
Fuel | Levelized Prices, as Applied in the WASP Model | Current Prices (Taka Prices Converted at 62Taka/US$) |
Natural gas | US$2.8707/MCF | US$1.1921/MCF |
Domestic coal | US$43.80/ton | US$55.00/ton |
Heavy Fuel oil | US$0.1595/liter | US$0.1934/liter |
High Sulfur Diesel | US$0.2346/liter | US$0.3608/liter |
8.2.1 Yearly Cash Flow Forecast to 2025
8.2.1.1 Overall Summary
Table 8-6 summarizes the cash flows required by PSMP, including both generation and transmission.
Table 8-6 Overall Summary of Cash Flows
Power Sector Master Plan - Cash Flows Figures in US$ millions, 2005 constant prices |
| Generation (Public/Private) | Transmission (Public) | Generation & Transmission |
Year Ending June 30 | Invest-ments Excl IDC | Fuel Costs at Level- ized Prices | Fuel Costs atCur- rent Prices | O&M Costs | Total | Invest- ments Excl IDC | O&M Costs | Total | Invest-ments Excl IDC | Opera- tional at Level ized Fuel Prices | Opera- tional at Cur- rent Fuel Prices | Total With Level- ized Fuel Prices | Total With Cur-rent Fuel Prices |
With Level- ized Fuel Prices | With Cur- rent Fuel Prices |
| | | | | | | | | | | | | | |
2005 | 248 | 702 | 340 | 124 | 1,074 | 712 | 84 | 14 | 99 | 332 | 840 | 478 | 1,173 | 811 |
2006 | 549 | 738 | 372 | 138 | 1,424 | 1,058 | 103 | 14 | 117 | 651 | 889 | 523 | 1,541 | 1,175 |
2007 | 896 | 811 | 405 | 157 | 1,864 | 1,457 | 239 | 18 | 257 | 1,135 | 986 | 579 | 2,121 | 1,715 |
2008 | 856 | 867 | 417 | 161 | 1,883 | 1,434 | 136 | 20 | 156 | 992 | 1,047 | 598 | 2,040 | 1,591 |
2009 | 468 | 924 | 426 | 180 | 1,571 | 1,073 | 51 | 21 | 72 | 519 | 1,124 | 626 | 1,643 | 1,145 |
2010 | 459 | 964 | 441 | 185 | 1,608 | 1,085 | 34 | 21 | 55 | 493 | 1,170 | 647 | 1,663 | 1,140 |
2011 | 394 | 1,036 | 471 | 193 | 1,623 | 1,058 | 35 | 22 | 57 | 429 | 1,251 | 686 | 1,680 | 1,115 |
2012 | 485 | 1,101 | 496 | 202 | 1,788 | 1,183 | 35 | 22 | 58 | 521 | 1,325 | 720 | 1,846 | 1,241 |
2013 | 550 | 1,177 | 529 | 210 | 1,937 | 1,289 | 35 | 23 | 58 | 586 | 1,410 | 761 | 1,996 | 1,347 |
2014 | 517 | 1,258 | 562 | 220 | 1,996 | 1,299 | 35 | 23 | 59 | 553 | 1,502 | 805 | 2,055 | 1,358 |
2015 | 285 | 1,347 | 589 | 232 | 1,864 | 1,106 | 35 | 24 | 59 | 321 | 1,603 | 844 | 1,923 | 1,165 |
2016 | 453 | 1,434 | 624 | 243 | 2,129 | 1,320 | 63 | 25 | 87 | 515 | 1,701 | 892 | 2,216 | 1,407 |
2017 | 507 | 1,569 | 681 | 256 | 2,331 | 1,443 | 63 | 26 | 88 | 570 | 1,850 | 962 | 2,420 | 1,532 |
2018 | 665 | 1,678 | 726 | 269 | 2,612 | 1,660 | 63 | 27 | 89 | 728 | 1,974 | 1,022 | 2,701 | 1,749 |
2019 | 546 | 1,583 | 677 | 273 | 2,402 | 1,496 | 63 | 28 | 90 | 609 | 1,884 | 977 | 2,493 | 1,586 |
2020 | 700 | 1,683 | 718 | 288 | 2,671 | 1,706 | 63 | 29 | 91 | 762 | 2,000 | 1,035 | 2,762 | 1,797 |
2021 | 719 | 1,767 | 753 | 294 | 2,780 | 1,766 | 29 | 29 | 59 | 749 | 2,090 | 1,076 | 2,838 | 1,825 |
2022 | 704 | 1,862 | 792 | 304 | 2,870 | 1,800 | 29 | 30 | 59 | 734 | 2,195 | 1,125 | 2,929 | 1,859 |
2023 | 494 | 1,968 | 836 | 316 | 2,778 | 1,646 | 29 | 30 | 59 | 523 | 2,314 | 1,182 | 2,837 | 1,705 |
2024 | 255 | 2,080 | 882 | 328 | 2,663 | 1,465 | 29 | 30 | 60 | 284 | 2,439 | 1,241 | 2,723 | 1,525 |
2025 | 0 | 2,210 | 936 | 346 | 2,556 | 1,282 | 29 | 31 | 60 | 29 | 2,587 | 1,313 | 2,617 | 1,343 |
| | | | | | | | | | | | | | |
Totals 2005-25 | 10,750 | 28,758 | 12,671 | 4,917 | 44,424 | 28,338 | 1,285 | 507 | 1,792 | 12,035 | 34,181 | 18,095 | 46,216 | 30,130 |
8.2.1.2 Generation Investments
Table 8-7 shows the build-up of the generation investment values.
Table 8-7 Generation Investments
Power Sector Master Plan - Capital Investments (Generation) Figures in US$ millions, constant prices |
| On-going & Committed | New Plants - On-going & Committed | New Plants - Planned | Total Investments |
Year ending June 30 | Public Sector | Private Sector | Public/Private | Public/Private |
Local | Foreign | Total | Local | Foreign | Total | Local | Foreign | Total | Local | Foreign | Total |
| | | | | | | | | | | | |
2005 | 84 | 92 | 176 | 22 | 51 | 72 | 0 | 0 | 0 | 105 | 143 | 248 |
2006 | 80 | 141 | 222 | 80 | 188 | 268 | 22 | 37 | 59 | 182 | 366 | 549 |
2007 | 147 | 210 | 357 | 77 | 181 | 258 | 107 | 174 | 281 | 332 | 564 | 896 |
2008 | 257 | 97 | 354 | 23 | 55 | 78 | 166 | 258 | 423 | 446 | 410 | 856 |
2009 | 41 | 33 | 74 | 0 | 0 | 0 | 153 | 240 | 394 | 195 | 273 | 468 |
2010 | 0 | 0 | 0 | 0 | 0 | 0 | 178 | 281 | 459 | 178 | 281 | 459 |
2011 | 0 | 0 | 0 | 0 | 0 | 0 | 153 | 240 | 394 | 153 | 240 | 394 |
2012 | 0 | 0 | 0 | 0 | 0 | 0 | 188 | 297 | 485 | 188 | 297 | 485 |
2013 | 0 | 0 | 0 | 0 | 0 | 0 | 215 | 336 | 550 | 215 | 336 | 550 |
2014 | 0 | 0 | 0 | 0 | 0 | 0 | 201 | 316 | 517 | 201 | 316 | 517 |
2015 | 0 | 0 | 0 | 0 | 0 | 0 | 107 | 178 | 285 | 107 | 178 | 285 |
2016 | 0 | 0 | 0 | 0 | 0 | 0 | 160 | 292 | 453 | 160 | 292 | 453 |
2017 | 0 | 0 | 0 | 0 | 0 | 0 | 181 | 326 | 507 | 181 | 326 | 507 |
2018 | 0 | 0 | 0 | 0 | 0 | 0 | 237 | 428 | 665 | 237 | 428 | 665 |
2019 | 0 | 0 | 0 | 0 | 0 | 0 | 195 | 351 | 546 | 195 | 351 | 546 |
2020 | 0 | 0 | 0 | 0 | 0 | 0 | 250 | 450 | 700 | 250 | 450 | 700 |
2021 | 0 | 0 | 0 | 0 | 0 | 0 | 258 | 461 | 719 | 258 | 461 | 719 |
2022 | 0 | 0 | 0 | 0 | 0 | 0 | 252 | 452 | 704 | 252 | 452 | 704 |
2023 | 0 | 0 | 0 | 0 | 0 | 0 | 176 | 317 | 494 | 176 | 317 | 494 |
2024 | 0 | 0 | 0 | 0 | 0 | 0 | 90 | 165 | 255 | 90 | 165 | 255 |
2025 | 0 | 0 | 0 | 0 | 0 | 0 | 0 | 0 | 0 | 0 | 0 | 0 |
| | | | | | | | | | | | |
Totals 2005-25 | 610 | 573 | 1,183 | 203 | 474 | 677 | 3,290 | 5,600 | 8,890 | 4,103 | 6,647 | 10,750 |
8.2.1.3 Transmission Investments
Table 8-8 shows the build-up of the transmission investment values.
Table 8-8 Transmission Investments
Power Sector Master Plan - Capital Investments (Transmission) Figures in US$ millions, constant prices |
Year ending June 30 | On-going & Committed | Planned Investments | Total Investments |
Public Sector | Public Sector | Public Sector |
Local | Foreign | Total | Local | Foreign | Total | Local | Foreign | Total |
| | | | | | | | | |
2005 | 34 | 50 | 84 | 0 | 0 | 0 | 34 | 50 | 84 |
2006 | 45 | 58 | 103 | 0 | 0 | 0 | 45 | 58 | 103 |
2007 | 73 | 132 | 205 | 12 | 22 | 34 | 85 | 154 | 239 |
2008 | 35 | 67 | 102 | 12 | 22 | 34 | 47 | 89 | 136 |
2009 | 6 | 11 | 17 | 12 | 22 | 34 | 19 | 32 | 51 |
2010 | 0 | 0 | 0 | 12 | 22 | 34 | 12 | 22 | 34 |
2011 | 0 | 0 | 0 | 13 | 23 | 35 | 13 | 23 | 35 |
2012 | 0 | 0 | 0 | 13 | 23 | 35 | 13 | 23 | 35 |
2013 | 0 | 0 | 0 | 13 | 23 | 35 | 13 | 23 | 35 |
2014 | 0 | 0 | 0 | 13 | 23 | 35 | 13 | 23 | 35 |
2015 | 0 | 0 | 0 | 13 | 23 | 35 | 13 | 23 | 35 |
2016 | 0 | 0 | 0 | 23 | 40 | 63 | 23 | 40 | 63 |
2017 | 0 | 0 | 0 | 23 | 40 | 63 | 23 | 40 | 63 |
2018 | 0 | 0 | 0 | 23 | 40 | 63 | 23 | 40 | 63 |
2019 | 0 | 0 | 0 | 23 | 40 | 63 | 23 | 40 | 63 |
2020 | 0 | 0 | 0 | 23 | 40 | 63 | 23 | 40 | 63 |
2021 | 0 | 0 | 0 | 11 | 19 | 29 | 11 | 19 | 29 |
2022 | 0 | 0 | 0 | 11 | 19 | 29 | 11 | 19 | 29 |
2023 | 0 | 0 | 0 | 11 | 19 | 29 | 11 | 19 | 29 |
2024 | 0 | 0 | 0 | 11 | 19 | 29 | 11 | 19 | 29 |
2025 | 0 | 0 | 0 | 11 | 19 | 29 | 11 | 19 | 29 |
| | | | | | | | | |
Totals 2005- 25 | 193 | 318 | 511 | 279 | 495 | 774 | 472 | 813 | 1,285 |
8.2.2 Conclusions and Recommendations
The generation and transmission investment requirements over the next twenty years are substantial, averaging US$2.7 billion for generation and US$0.3 billion for transmission every five years. In order to meet the projected electricity demand, the necessary investments will need to be implemented in a timely manner. This will require careful planning and timely resource mobilization, both public and private. Table 8-9 shows that investment requirements over the next five years to June 2010 are estimated at US$3.2 billion for generation and US$0.6 billion for transmission.
Table 8-9 Investment Requirements July 2005 through June 2010
| Generation Public & Private | Transmission Public | Total Public (G&T) |
| US$ millions |
On-going And Committed | | | |
GOB/Internal Cash Generation | 526 | 159 | 685 |
Donors | 481 | 268 | 749 |
Private | 604 | - | - |
Total | 1,611 | 427 | 1,434 |
Planned (PSMP)* | | | |
GOB/Internal Cash Generation | 313 | 49 | 362 |
Donors | 495 | 87 | 582 |
Private | 808 | - | - |
Total | 1,616 | 136 | 944 |
Total (2005/06-2009/10) | | | |
GOB/Internal Cash Generation | 839 | 208 | 1,047 |
Donors | 976 | 355 | 1,331 |
Private | 1,412 | - | - |
Total | 3,227 | 563 | 2,378 |
* Planned investments (PSMP) - assuming 50% public sector & 50% private sector for generation investments |
The investment plan over the next five years is quite ambitious, requiring US$1.047 billion in GOB budgetary support and funding from internal cash generation of BPDB and PGCB, US$0.582 billion in new funding support from donors, and US$0.808 billion in “new” private sector investment. Based on existing tariffs, operational performance, and DESA’s payment record of its import energy bills from BPDB, investment funding from internal cash generation of BPDB and PGCB will be minimal and, consequently the burden on the central treasury will be substantial. The annual average support from GOB over the next five years for generation and transmission investments (excluding distribution) will be in the region of US$200 million, compared to the annual average of approximately US$100 million during 2003/04 and 2002/03. An increase of 100% in annual GOB support may not be sustainable in the medium to long-term, and donors may be reluctant to provide further support unless concrete steps are taken to achieve and sustain the financial viability of the power sector. This implies further efficiency improvements and a phased tariff adjustment implementation plan that will lead to cost reflective tariffs over the next two to three years. Ultimately, electricity tariffs should be adequate for the power utilities to meet at least 20% of their investment requirements from internal cash generation.
The on-going and committed generation and transmission projects of BPDB and PGCB will require increasingly larger capital contributions (loan and equity) from GOB in the coming two to three years. The projected capital injection from GOB will grow from US$125 million in 2005/06 to US$220 million in 2006/07 and US$292 million in 2007/08; such burdens on the central treasury may be unsustainable. In addition, funding of foreign currency costs have yet to be secured from external lenders for some of these projects, and appraisal and financial closures on some of the “agreed in principle” projects, such as the ADB and WB funded 3*120MW peaking plants at Siddhirganj, may take longer than envisaged. For these reasons, there may be slippages in the projected commissioning dates of the on-going and committed new generating plants, leading to capacity shortages in the short to medium-term. This is a cause for concern and we recommend that a realistic investment plan concerning these generating plants is drawn up by BPDB, in consultation with the Government and donors. In parallel, a realistic energy supply/demand balance needs to be prepared for the next two to three years.